Environmental Science for Environmental Sustainability
Environmental science is the dynamic, interdisciplinary study of the interaction of living and non-living parts of the environment, with a special focus on the impact of humans on the environment. Environmental science studies include circumstances, objects, or conditions surrounding an organism or community and their complex interactions. Our future depends on our ability to understand and evaluate evidence-based arguments about the environmental consequences of human actions and technologies and to make informed decisions based on those arguments – environmental science provides much of the evidence needed.
From global climate change to habitat loss driven by human population growth and development, Earth is becoming a different planet—right before our eyes. The global scale and rate of environmental change are beyond anything in recorded human history. Our challenge is to understand better Earth’s complex environmental systems, systems characterized by interactions within and among their natural and human components that link local to global and short-term to long-term phenomena, and individual behavior to collective action. The complexity of environmental challenges demands that we all participate in finding and implementing solutions leading to long-term environmental sustainability.
In this chapter, we’ll gather some basics – a general understanding of how energy and temperature move around the planet and a bit of chemistry, so we can talk about physical stuff, too.
Energy, Temperature, and a Spinning Planet – the Climate System
Our environmental problems take place on a planet with lots of moving parts. Some of our environmental problems (air pollution, for example) hitch rides on the moving parts! So as part of our introduction, we need to know the baseline moving parts and the forces that drive them and that move with them.
First, three videos from the UK meteorological service (Met Office) that provide helpful visual support for understanding how air and temperature move on a spinning planet.
How does the climate system work?
What is global circulation – the three cells
What is global circulation – the Coriolis effect & winds
The combination of waters of different temperature and salinity, plus the Coriolis effect give rise to the global conveyor belt of ocean currents also known as the Meridional Overturning Circulation (MOC) This conveyor belt distributes warm water away from the equator and helps to moderate ocean temperatures in temperate and polar latitudes, just as the atmospheric cells move warm air away from the equator into cooler latitudes. The Atlantic portion of this pattern is important enough to the Northern Hemisphere nations around the Atlantic that is is often discussed separately, as the Atlantic meridional overturning circulation, or AMOC. This video from the PBS NOVA collection explains the basics of global ocean circulation.
Chemistry for Understanding Environmental Sustainability
This section is designed for readers with no background in chemistry, but may serve as a useful review for those who do have some background. It is not designed to teach chemistry, but to provide a level of comfort in reading about chemistry as it relates to environmental sustainability. Because of this focus, it approaches chemistry differently than traditional chemistry instruction. The tone is occasionally somewhat irreverent, to offset the potentially off-putting nature of the subject material.
First – atoms and molecules
Atoms are the basic unit of chemistry – the smallest bits of single chemical elements. Atoms combine to form molecules that may comprise a single chemical element (for example, O2 or N2 – oxygen and nitrogen as they exist in their usual form as gases in the atmosphere), just two elements (water, H2O is well-known example) or several (for example, glucose, a simple sugar, which is written C6H12O6. Atomic abbreviations come from the periodic table (Fig 1)- a formal presentation of all the elements known to science. As of early 2025, there are 118 chemical elements formally described to science, but several of these only exist when researchers undertake rather violent experiments in carefully controlled laboratory conditions, and many more are uncommon. Many of the abbreviations seem very sensible: C for carbon, O for oxygen, H for hydrogen, He for helium, Al for aluminum, and so forth. Others seem very odd: Fe for iron, Na for sodium, K for potassium, for example. Most of the oddballs were discovered rather early, when Latin was still an important language for science, and reflect Latin names: ferrum, natrium, and kalium for these examples. Note that whereas the abbreviations for the names of elements are capitalized (being abbreviations), the names of the elements themselves are not capitalized.
The periodic table groups similar kinds of elements – several different kinds of metals, for example, or the unreactive chemicals called the noble gases that don’t participate in chemical reactions. These groups have somewhat similar chemistries, and their behaviors can be predicted, to some extent, by their membership in these groups.
Column VIIA of the periodic table contains the elements called halogens. Only chlorine through iodine occur with regularity. Chlorine (Cl), fluorine (F), and bromine (Br) are all toxic. But chemists find them useful and use them to create compounds of many kinds, many of which have become environmental problems due to toxicity. The words halogenated , chlorinated, fluorinated, or brominated signal a potentially harmful compound, particularly if it’s a synthetic organic compound. But note that plenty of fairly harmless compounds, including table salt (sodium chloride – NaCl) also contain halogens.
Atoms combine to form molecules because atoms have electrical charges that attract them to each other (forming ionic bonds) or because they become more stable in the combined state (forming covalent bonds). Some can attract themselves – O2 and N2 are gaseous examples and soot, graphite and diamond are all solid examples of pure C – but other elements never occur in their pure form in nature. Iron occurs primarily with oxygen in ores such as hematite, Fe2O3, and magnetite, Fe3O4. Some of the bonds between atoms are easily undone in certain circumstances – table salt, NaCl, and many other ionically-bonded compounds dissolve (dissociate, technically) easily in water. Table salt dissociates to leave charged ions of sodium (Na+) and chlorine (Cl–) separately floating around. Adding energy through sunlight or heat can break covalent bonds. For example, sunlight is an important part of the atmospheric chemistry that creates smog. Fire can break the bonds of lignin, the very large molecule that is the basis for wood, turning it into carbon dioxide and water.
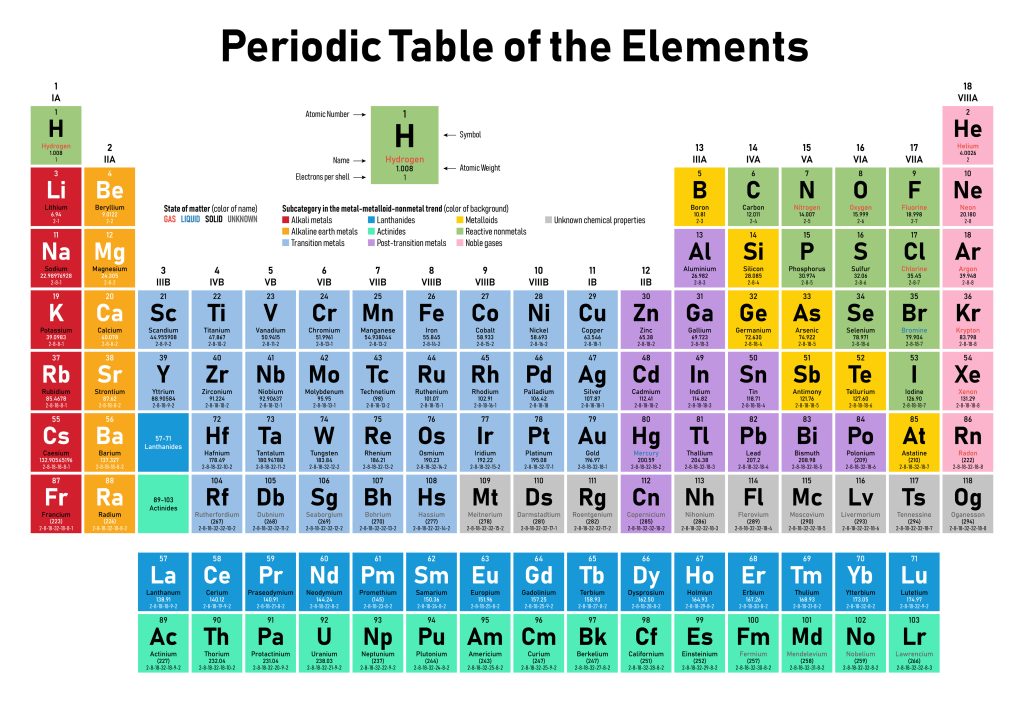
Figure 1. The periodic table of the elements. Each cell provides information on a single chemical element. Elements are grouped into subsets (shown here by the color of the cell) that provide insight into their chemical behavior.
Elements for Life
Living things are composed mostly of carbohydrates, fats, and proteins. Carbohydrates and fats are made up of carbon, hydrogen, and oxygen. Lignin, from which wood is built, needs only C, H, and O. Proteins all add nitrogen. Fur, made of keratin is made of the same amino acids that make up protein, and requires only C, H, O, and N. More complicated proteins add sulfur (S) which keeps proteins folded into their proper shapes. Some proteins add phosphorus (P) and a variety of trace amounts of other elements. Bones and teeth need calcium (Ca) and phosphorus. Enzymes, which serve as catalysts to help chemistry happen quickly in living things, use a variety of trace elements, including several metals: zinc (Zi), selenium (Se), copper (Cu – cuprum), manganese (Mn), iron, cobalt (Co), molybdenum (Mo), and nickel (Ni). Very small quantities of elements can be hugely important: a single atom of magnesium (Mg) is at the heart of chlorophyll – the green stuff that makes photosynthesis (and most of life) possible – and four atoms of iron (Fe) occur in hemoglobin – the stuff that makes blood red and that captures oxygen in our lungs and allows it to be delivered throughout our bodies, far from the atmosphere. But C, H, O, and N make up more than 90% of the weight of all living things.
Most of the compounds in living things are organic compounds – compounds built on a backbone of carbon atoms in chains and rings (Fig 2, 3). The simplest organic compounds, simple hydrocarbons composed only of C and H, are not found in living things but derive from components of living things. They are the found in fossil fuels components: methane (CH4, also called natural gas or swamp gas) and ethane (C2H6) (Fig 2). Remember that fossil fuels resulted from buried organic material – mostly buried vegetation – subjected to heat and pressure over geological time. No surprise, then, that fossil fuels should be a complicated mix of organic compounds. Plastics are an example of anthropogenic (human-made) organic materials, generally created from fossil fuels. Many other synthetic chemical compounds, including important hazardous substances are also compounds.
Figure 2. Simple organic molecules. Top row from left: methane, ethane, methanol. Bottom row from left: cyclohexane, benzene. Images built with MolView.
The 3-D molecular models built here show carbon atoms in gray, hydrogen in white, and oxygen in red. Each carbon atom can form four bonds. Methane and ethane are the two simplest hydrocarbons (molecules that contain only C and H), with one and two carbons, respectively. Methanol is the simplest alcohol, with a single carbon. The OH group (the red atom connected to the white atom) makes it an alcohol. The lower row shows two hydrocarbon ring structures, each with 6 carbons but different numbers of hydrogen atoms. Cyclohexane has single bonds between the carbon atoms, leaving each C with 2 bonds to share with hydrogen atoms. Benzene has double bonds between every other pair of carbon atoms, so each C atom has only a single bond left to share with hydrogen.
Figure 3. More complex organic molecules. Top: glucose, starch. Bottom: sulfanilamide, chlorophyll. The carbon chains and rings are in gray. Images built with MolView.
Glucose is the simplest sugar, and the simplest carbohydrate. It has a ring structure that includes an O molecule, which has two bonds available. Starch is a more complex carbohydrate. Both glucose and starch, being carbohydrates, have only C, H, and O atoms. Sulfanilamide is a common antibiotic with a benzene ring in it, along with N atoms (blue) and a S atom (yellow). Chlorophyll contains a long, branched hydrocarbon “tail,” and a more complex structure that includes rings, 4 nitrogen atoms (blue) and a single magnesium atom (green).
The phrase persistent organic pollutants refers to synthetic organic compounds that that may take decades or centuries to be broken down, and that can continue to harm living things during that time. “Forever chemicals” is a newer, more emotional phrase that describes the same phenomenon. Keep in mind that naturally occurring, but toxic elements such as lead, mercury, and other heavy metals cannot ever break down because they are already simply elements.
Some persistent pollutants, including heavy metals, avoid being broken down by digestion when they enter the bodies of organisms (from plankton to polar bears) through contaminated food and water. Instead, the body may treat them as fats and store them in fat deposits, in the case of organic pollutants or use them in place of useful elements such as calcium, in the case of heavy metals. Plankton in contaminated water are tiny and likely only hold a tiny amount of the contaminant. But over the short lifetime of the plankton, it will continue to take in more of the contaminant and will not lose most of it. This gradual build-up of a toxin over the lifetime of an organism is termed bioaccumulation. Fish that eat the plankton will also retain the contaminant, and will bioaccumulate more over their lives, eating many plankton each day. Because they eat lots of plankton, the level of toxin in the fish is much higher than the level in the plankton – termed biomagnification or bioamplification. (Fig 4). As organisms at each successive level of a food chain encounters the toxin, the levels of toxin rise sharply, and the likelihood of harm increases. Different toxins cause different kinds of harm – neurological or developmental damage, for example. Birds of prey and other predators, along with humans, who also eat at the tops of some food chains, are at most risk or harm. Persistent organic pollutants but also some inorganic toxins such as heavy metals may bioaccumulate and biomagnify.
One class forever chemicals has become a particular focus in the 2010s and 2020s: PFAS – perfluorylalkyl and polyfluorylalkyl substances. Thousands of these compounds exist; they have been in use since the 1930s and 1940s and are now widely dispersed throughout the world in all ecosystems and all human habitations. They are responsible for a wide range of health impacts including cancer, endocrine disruption, developmental issues, and immune deficiencies. Regulation of PFAS in drinking water only began in 2024.
Inorganic compounds – compounds that those not based on carbon chains and rings – are also important. Salts, metals, ores, crystals, and all the other forms of minerals are inorganic.
When we discuss soil, we divide it into its mineral components, which derive from the breakdown of rock – sand, silt, and clay – and its organic components that derive from the breakdown of living things. Good topsoil, for agricultural purposes, has 3-6% organic material by weight, along with about 45% mineral components; 50% is pore space containing water and air. Steel and concrete – vital for construction – are entirely inorganic, as are metal alloys like the metal in aluminum cans and automobile components, glass, and porcelain.
Asphalt, used for paving roads, contains components derived from fossil fuels mixed with mineral components – it is a mix of both organic and inorganic material. Such mixes occur naturally as well, for example, in bones and teeth. A number of grasses incorporate silica (silicon dioxide: SiO2 – in larger amounts it is called sand) into their leaves to make their tissues more resistant to grazing and digestion, to deter herbivores.
Elements of Air
Earth’s atmosphere has changed immensely over the history of the planet. The early atmosphere would have been poisonous to most present life forms, and lacked oxygen. Today, earth’s atmosphere is dominated by nitrogen (78%) and oxygen (21%). The remaining gases, including carbon dioxide (CO2), methane (CH4), water vapor, and other trace gases, compose only 1% of the atmosphere but include the gases that regulate planetary temperatures and affect human and environmental health. Volcanos, which contributed much of the gas volume for the early Earth atmosphere, can change the balance of trace gases in the present, during eruptions.
The oxygen in our present atmosphere is created by plants, which produce oxygen as a byproduct of photosynthesis – the process of using light energy to combine water and CO2 to produce sugar – that is the basis for all food webs (almost all – a few microorganisms do really interesting things with chemistry, instead). Plants and animals take in oxygen to fuel metabolism – the process that maintains, builds, and repairs organisms – and all these organisms produce CO2 as a result of metabolic processes. Plant biomass is approximately 180 times the animal biomass, and plants undertake photosynthesis, so they are the major players in this O2 – CO2 swapping process.
Greenhouse gases (GHG) are atmospheric gases that absorb heat radiated by Earth, and re-radiate some of it back to the planet. Greenhouse gases are the reason that Earth has temperatures that are much milder than temperature on the Moon, which is the same average distance from the sun as Earth. Without greenhouse gases, life on Earth would not be possible. Water vapor is the primary greenhouse gas in our atmosphere, but because humans do not directly affect the concentration of water vapor in the atmosphere, it is not a target for control. Carbon dioxide, methane (swamp gas, natural gas), and nitrous oxide (N2O) are the main anthropogenic greenhouse gases. We will see more of them in the next section.
In the stratosphere, one of the higher atmospheric layers well above the Earth’s surface, the sun’s energy is strong enough to split oxygen molecules, which then interact with unsplit molecules to form a layer of ozone (O3), a highly reactive, short-lived compound that absorbs ultraviolet (UV) radiation. This stratospheric ozone layer is important for protecting life on Earth from heavy doses of UV that could damage DNA, harm exposed body surfaces and vegetation, and cause cancers. Air pollution can thin the ozone layer, creating health risks and other environmental harm.
Acids, Bases, and pH
Acids and bases are part of a larger group of hazardous materials that are corrosive – that can chemically attack other compounds, chemically burning them and breaking them down. In nature, acidic compounds are more common than basic compounds and can be more corrosive.
Acids and bases can exist in solid, liquid, or gaseous form, but in the environment, water-based forms are most common. Familiar acids include sulfuric acid (H2SO4 – a component of acid rain), hydrochloric acid (HCl – a component of stomach acid) and acetic acid (CH3COOH; vinegar – an organic acid). Familiar bases include lye (NaOH), ammonia (NH3), and baking soda (NaHCO3 – often used to treat an acid stomach). Acids and bases neutralize each other, with acids donating hydrogen ions (H+) and bases accepting them. If you spill acid, you can limit the damage by adding a base, and vice versa.
The measurement of the strength of acids is not completely straightforward, but it’s not rocket science, either. Bear with me. Because hydrogen ions are a major part of the chemistry, their concentration is the quantity that is measured. pH stands for the potential of hydrogen; it is always written with a lower-case ‘p’ and an upper-case ‘H’.
Molecules in water tend to fall apart (dissociate) a bit, so there’s always some hydrogen and hydroxide ions (H+ and OH–) in the solution, with a concentration of hydrogen ions of 10-7 moles/liter of solution. [Detour: in chemistry, moles are not small mammals, but rather an amount equal to the molecular weight of the substance (for practical purposes). Hydrogen has a molecular weight of 1 gram/mole, so pure water has 10-7 grams of hydrogen ions per liter of water.]
Because acids dissolved in water produce hydrogen ions, acid solutions have more hydrogen ions than pure water. The pH scale measures hydrogen ions as the negative logarithm (in base 10) of the concentration of hydrogen ions. Don’t gibber. It’s not that bad. The negative logarithm of 10-7 is 7. So the pH of pure water – a neutral substance – is 7. More hydrogen ions means a higher concentration – perhaps 10-5. The pH of that solution would be 5. Each unit of pH is an order of magnitude – a multiplication by 10. So a pH of 5 is 100 times more concentration than a pH of 7.
The pH scale is neutral at 7, acid at less than 7, and basic at greater than 7. Rainwater, which contains some carbonic acid (H2CO3) due to dissolved CO2, has a pH around 5.5, which is 101.5 or 31.6 times more concentrated than neutral. Stomach acid in humans has a pH of about 2 – very corrosive – but the stomach acid of vultures is near 0 – 100 times stronger than humans, and suitable for destroying bacteria and disease organisms in their food.
Basic substances have pH values between 7 and 14. The pH of blood is between 7.35 and 7.45 – just slightly basic. Soils that form over limestone have pH values of 7.5-8, still only one pH unit from neutral. Seawater has a pH of 8.1. A baking soda solution can reach pH 9. The most basic, naturally occurring liquid I can find in the literature would be produced when a volcano superheats limestone (calcium carbonate – CaCO3), creating calcium oxide (CaO), which dissolves in water to create Ca(OH)2 – calcium hydroxide. Note the similarity to lye, which is sodium hydroxide – NaOH. The pH of the strongest possible concentration of calcium hydroxide would be about 12.4. But you need a volcano to do it. Natural, but not common! And not associated with living things.
Figure 4. The pH scale indicates the concentration of hydrogen ions on a scale ranging from very acidic (pH 0) to very basic (pH 14). Pure water, which is neutral, has a pH value of 7.
Limiting substances
Limiting substances are a concept at the interface between chemistry and biology. Living things take in nutrients – by eating food if they are animals or by taking up dissolved materials through their roots if they are plants. If animals don’t get enough calories or plants don’t get enough light (which they use for photosynthesis, which allows them to create carbohydrates), they eventually die. If the diet provides enough energy but does not contain nutrients in the proportions that are needed, then deficiencies can slow growth and harm health.
The idea of limiting substances is most often applied to plants, including algae. Because plants can photosynthesize and create carbohydrates, they can generally have enough C, H, and O. Nitrogen and phosphorus are important nutrients that they cannot make for themselves and must absorb from the soil. In situations where a unit of added nitrogen produces more plant growth than a unit of added phosphorus, then nitrogen is the limiting substance. If a unit of added phosphorus produces more growth, then it is the limiting substance. These two nutrients are most often limiting, but under some circumstances, other nutrients can be involved, and occasionally, too much of a nutrient (particularly trace elements such as selenium – Se) can be a greater problem, acting as a poison.
In most terrestrial ecosystems, and in freshwater, N is the limiting substance for plant and algal growth, although P will likely also increase growth, to a lesser extent. In saltwater, P is the limiting substance. The issue of N or P limitations will come up when we look at water pollution, because nutrient pollution from fertilizer runoff from agricultural lands, from manure runoff from grazing lands and confined-animal feeding operations, and sewage from municipal water treatment can all change the nutrient balance in receiving waters, leading to nutrient pollution. Knowing which nutrient is limiting can suggest efficient ways of dealing with the problem.
“Natural” does not mean “beneficial to humans;” “synthetic” does not mean “harmful to humans.”
One final note here that applies particularly to compounds but also to other environmental factors. The natural world, as you likely have noticed, is not composed solely of puppies, butterflies, and rainbows. Its substances and phenomena can be powerfully harmful. The stomach acid of vultures is approximately the pH of battery acid. We use the venoms of animals and poisons of plants (if they bite you and you die, they are venomous; if you bite them and you die, they are poisonous) to create many products – some help individuals (pain relievers, for example) while others are weapons (mustard gas and ricin, e.g.). Vultures benefit from their strong stomachs and plants and animals benefit from their offensive and defensive chemicals, but that doesn’t mean humans can encounter these substances without harm.
Disease organisms that have killed large portions of human populations arose naturally, although they can also be weaponized. Vaccines created to combat disease are often merely weakened forms of those organisms and are very helpful.
On the other side, synthetic substances created in laboratories also cannot easily be categorized. Plastics are becoming a major environmental problem not merely from the mass of waste that has accumulated, but also because they break down into microplastics that are increasingly seen as a health hazard to organisms of all kinds. However, plastics have also improved quality of life for many people through inexpensive clothing, lower costs for shipping due to light-weight containers, safer, more nutritious food due to impermeable wrappings, medical appliances that depend on the properties of particular plastics, etc. As in most undertakings, we can make the best progress towards sustainability using decisions based on careful analysis and thorough information.
Media Attributions
- Colorful Periodic Table of the Elements – shows atomic number, symbol, name, atomic weight, electrons per shell, state of matter and element category
- Simple molecules
- complex molecules
- Bioaccumulation vs biomagnification toxic poisoning process outline diagram
- acid-base bar