7 Molecular Geometry and Polarity
Purpose
To study the molecular geometry and polarity of a range of molecules.
Expected Learning Outcomes
After completing this experiment, you should be able to:
- Determine the Lewis structure, molecular geometry and polarity for molecules using Lewis structures.
- Sketch molecular geometries on paper with wedges and dashes to represent their 3D shape.
- Explain how π-bonding affects the ability of atoms to rotate about a bond.
- Predict the bond dipoles and partial charges on a molecule and use them to determine the molecular polarity of that molecule.
Textbook Reference
Tro, Chemistry: Structures and Properties, Ch. 5.
Introduction
Life would be very boring if we tried to live with elements alone. We have 92 naturally occurring (plus a number of artificially made) elements, and that’s it. The paucity of materials with which we can live with based on elements alone is beyond imagination. In reality, we have many different types of compounds, each with distinct structures.
There are different types of chemical bonding involved in making compounds. In this experiment, we focus on molecular compounds, which are held together by covalent bonds.[1]
Covalent bonds are formed when electrons are shared between the atoms in a molecule. Depending on the bonding pattern about each of the different central atoms in a molecule, each molecule will have a different 3D structure – the molecular geometry. The bonding pattern is the Lewis structure.
The molecular geometry has a direct impact on the compound’s physical properties and chemical reactivity. The molecular geometry of a species affects crystal structure, phase change behavior, energy and rates of chemical interactions, solubility, and virtually every other characteristic of that compound.
Before beginning to study the geometries of covalently-bonded groups, it is necessary to have a clear picture of which atoms are present and which atoms are bonded directly together in the grouping. The flow chart below gives a schematic view for how one can figure out the molecular geometry from the molecular formula:
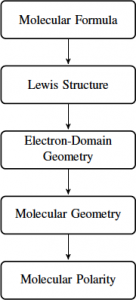
You cannot skip steps on this process.
Determining the Lewis Structure
While there are a number of different ways of determining the Lewis structure, the basic principles remain the same:
- All valence electrons must be found either in a bonding pair or in a non-bonding pair (lone pair).
- The starting number of valence electrons for an atom is equal to the group number.
- Most of the time, there will be a full valence shell of electrons around each atom. This is called the octet rule, because the final number of valence electrons around most atoms is eight. For this purpose, hydrogen is treated as an exception; hydrogen requires two valence electrons to fulfill the octet rule.
Another couple of useful guidelines are that, for many non-metal elements, the number of covalent bonds is equal to [latex](8-[\mbox{group number}))[/latex], as listed in the table below. The rest of the octet will be completed with lone pairs.
Group | Common Elements | No. of Bonds | No. of Lone Pairs |
H | 1 | 0 | |
4A | C, Si | 4 | 0 |
5A | N, P, As | 3 | 1 |
6A | O, S, Se | 2 | 2 |
7A | F, Cl, Br, I | 1 | 3 |
Every electron that is either in a lone pair on a given atom, or in a bonding pair where one end of that bonding pair is on that atom, is counted towards that atom for the purpose of the octet rule. Also, there are many exceptions to these rules, so only treat them as a starting point.
Exceptions to the Octet Rule
The octet rule is not a fundamental rule – it’s just that we try to draw Lewis structures that do not violate them (and that do not have large non-zero formal charges). The most fundamental rule is that all electrons must be accounted for. As explained in Tro, Chemistry: Structures and Properties, 2nd Ed, Ch. 5.5, there are three common reasons why the octet rule is violated:
- Radicals: There are an odd number of electrons.
- Incomplete octet: There is less than an octet of electrons around the central atom. This happens most frequently with beryllium (Group II), aluminum and boron (Group III) compounds.
- Expanded octet: In periods 3 and below, where the valence shell has d orbitals available, one can have greater than an octet about a central atom.
Determining Electron Geometries and Molecular Geometries
Based on the valence bond electron pair repulsion (VSEPR) theory, molecules have a particular geometry depending on the number and identities of electron groups[2] present around each central atom. To count the number of electron groups:
- Each non-bonding pair is a single electron group.
- Each set of bonds – whether it is triple, double or single – each counts as one electron group.
Examples
Formaldehyde has, around its central carbon atom, two single bonds (to hydrogen) and one double bonds (to oxygen):
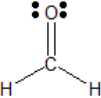
Each set of bonds would count as a single electron group, and therefore there are three electron groups about the central carbon atom.
Based on this, we can determine the electron geometry (i.e. how the electrons are arranged around the central atom) by comparing it with a set of standard geometries that are based on the idea that electron pairs repel each other and take on the geometry that minimizes their repulsion:
However, instead of looking at the arrangement of electrons around a central atom (i.e. the electronic geometry), it is sometimes more useful to look at the arrangement of atoms around a central atom. This is known as the molecular geometry of the central atom. This is because some atoms have lone pairs, and while lone pairs participate in electron pair repulsion, thus they affect the arrangement of atoms in space, lone pairs are not atoms, thus they are not considered part of the molecular geometry itself. The molecular geometries that arise when lone pairs are present on an atom are listed in the table below:
If you get nothing else from this section of the manual, please remember this: electronic geometry is the shape of electrons around a central atom while molecular geometry is the shape of atoms around a central atom. For electronic geometry, ask yourself where the electrons are, regardless of whether those electrons are part of a bond or a lone pair. For molecular geometry, first figure out the electronic geometry, then look at where the atoms are, ignoring all electrons completely.
In this experiment, you will build models that illustrate these different molecular geometries. Another way to visualize these is to use the PhET Molecular Shapes animation.
For molecules with multiple central atoms, you should determine the geometry about each individual central atom.
Examples
Consider the structure of propene:
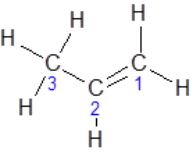
- About carbon atoms 1 and 2, there are three electron groups about each of them, and all of them are bonding pairs. As a result, the geometry is trigonal planar
- About carbon atom 3 there are four single bonds – and hence there are four electron groups. As a result, it is tetrahedral.
Drawing Molecules
Clearly, molecules have a 3D shape. However, it is not practical to pass around 3D models all the time (or make computer generated models). We therefore need to find a way to represent molecular geometries in 3D on 2D paper (or plain computer screens).
To draw molecules showing the 3D structure:
- Place as many bonds as possible on the plane of the paper. These are represented by normal straight lines.
- To draw bonds that project outwards from the paper, you will need to draw a solid wedge coming out.
- Conversely, if the bond goes into the paper, draw a dashed/hashed wedge instead.
For example, if we want to draw methane (CH4), it has 4 C-H single bonds, giving it tetrahedral molecular (and electronic) geometry. This means that methane’s bond angles are ~109.5°, not 90° the way it is often depicted with a Lewis structure. To be tetrahedral, not all of the H’s in methane can be in the same plane as the C. This means at least some of the C-H bonds need to be on wedges/dashes to indicate that they are in front of/behind the central C. This can also be seen in the 3-dimensional model of methane where the H (white sphere) circled in blue is shown farther back than the C (black sphere) (i.e. that H is behind the plane of the screen while the C is in the plane of the screen) while the H circled in orange is shown protruding out of the screen and thus is closer to you than the C is (i.e. that H is in front of the plane of the screen). Also note how the two C-H bonds that are on lines are NOT at a 90° angle. They are at ~109.5°. If you want to accurately depict the molecular geometry using wedges and dashes, make sure you accurately depict the bond angles everywhere on the molecule. If you would like to play with a 3D model of methane yourself to better understand what this is trying to show, go here.
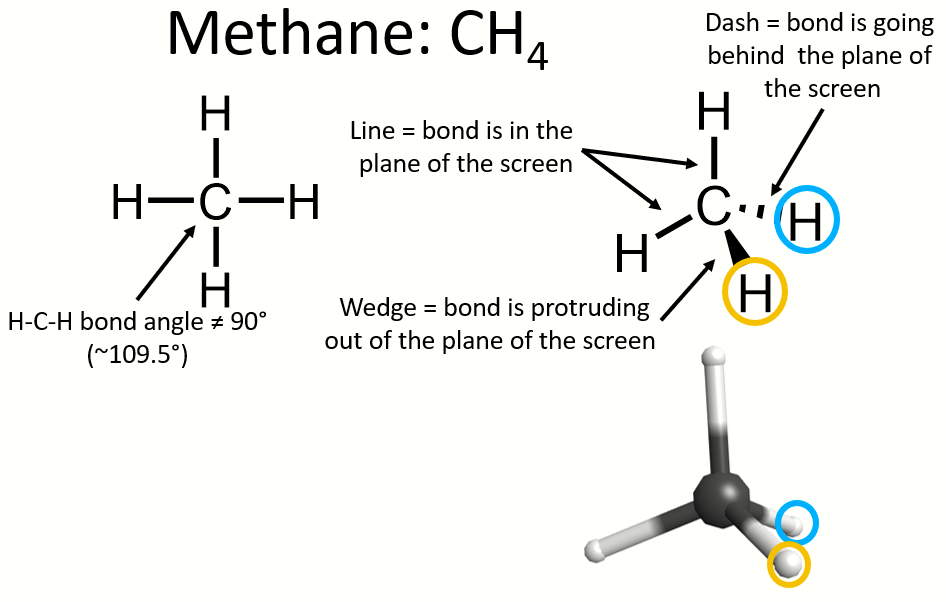
More examples of this can be seen in the tables above.
Molecular Polarity
Electronegativity and Bond Polarity
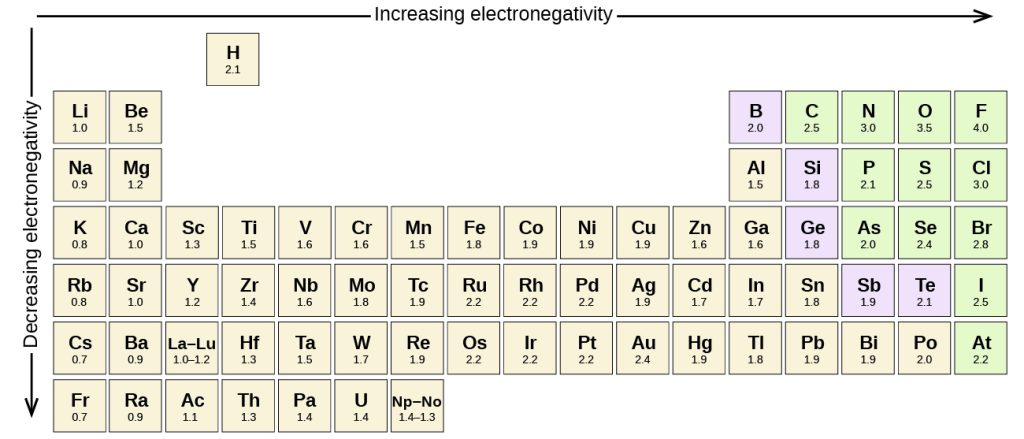
As you may have seen, depending on the difference in electronegativities of the different atoms in a bond, there would be a difference in the amount of electron density from a particular covalent bond that is placed on a particular atom.
Defining the electronegativity as the “ability of an atom to draw electron density from a covalent bond into itself” (Burdge and Overby, Chemistry: Atoms First), we can find bonds in a continuum from being pure covalent bonds to ionic bonds:
Depending on the difference in electronegativities in the two atoms involved, bonds can be classified as non-polar covalent, polar covalent or ionic as illustrated above. However, in practical terms it is important to have a “feel” for this, especially because the exact electronegativity value for each element can be slightly different depending on which source you use and because the cutoffs for what’s considered a non-polar vs polar vs ionic bond vary based on the source as well. A good touchstone for this is to remember that C-H bonds are always considered to be non-polar bonds. It’s also important to understand conceptually what each of these classifications mean.
- In a pure covalent bond, the electrons in the bond spend roughly half of their time on 1 atom and half their time on the other atom.
- In a polar covalent bond, the electrons in the bond spend significantly more time on 1 atom than the other atom (how much more time depends on the difference in electronegativities).
- In an ionic bond, the electrons spend all their time on 1 atom (the negatively charged anion atom) and none of their time on the other atom (the positively charged cation).
Examples
Due to the negative charge of electrons, the chlorine atom would have a partial negative charge (δ-), and correspondingly the hydrogen atom would have a partial positive charge (δ+). From the physics, this gives rise to a bond dipole moment running from the partial positive charge to the partial negative charge. To see a demonstration of how electronegativity affects bond dipoles and partial charges, check out this PhET simulation and select “Two Atoms”.
From Bond Polarity to Molecular Polarity
So far, we’ve focused on one bond between two atoms. However, most molecules have more than two atoms
It’s clear that if a molecule has no polar bonds, there is no significant separation of charge across the molecule since all of the electrons in the bonds are shared equally and therefore the molecule would not be polar. However, if one or more of the bonds are polar, the picture becomes more complex. Let’s take two different molecules – H2O (water) and CO2 (carbon dioxide) – and see how they can be different. In both cases, the bonds (H-O in the case of water and C=O in the case of carbon dioxide) are clearly polar – with the oxygen having the partial negative charge because it is more electronegative than C or H. This means that the electrons in the C=O bonds and H-O bonds spend more time on the O than on the C/H, leaving the O atoms with partial negative charges and the C/H’s with partial positive charges.
Water
In the case of water, you can see that in the vertical direction the partial positive charges (hydrogen atoms) are all closer to the bottom, while the partial negative charges (from the oxygen) closer to the top. In this case, due to the overall charge imbalance, the molecule is polar because the center of the partial positive charges on the molecule (half-way between the 2 H’s) is in a different spot than the center of the partial negative charge (the O atom since it’s the only atom with a partial negative charge in water).
Carbon Dioxide
On the other hand, in carbon dioxide the negative charges are equidistant and directly opposite each other along the O=C=O axis. In this case, we find that the two bond dipoles mathematically cancel out when determining the molecular dipole – while internally there is charge imbalance (the O’s have partial negative charges and the C has a partial positive charge), they mathematically cancel each other out when determining the molecular dipole (the bond dipoles still exist) and thus the center of the partial negative charge (half-way between the O atoms) is at the same place as the center of the partial positive charge (the C atom). This means we can’t say that any particular end of the molecule has an overall partial charge that is opposite to the other end. Therefore, if the molecule is symmetrical, then the molecule must be non-polar. You can check this out more by going to this PhET simulation and clicking on “Three Atoms” moving atom A around by clicking and dragging on it.
Summary
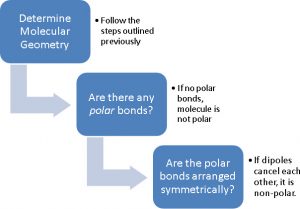
Application of Molecular Polarity to Intermolecular Forces
A particular application of molecular polarity is that, due to the opposing partial charges, opposite-charged poles on different molecules of the substance will attract each other. This is referred to as the dipole-dipole force.
Due to the presence of dipole-dipole forces, polar molecules have stronger intermolecular attraction with each other compared to non-polar molecules (provided that their molar masses are similar). This influences bulk properties of molecules such as melting and boiling points as well as its vapor pressure. This will be discussed in more detail in Tro, Chemistry – Structures and Properties, 2nd Ed, Chapter 11.
In addition, there are certain electrostatic properties associated with polar molecules which are absent with non-polar molecules. For example:
Examples
- Polar molecules will have attractive forces with opposite charges (and vice versa). This can be observed by running a liquid against a charged rod.
- Polar molecules tend to be more soluble in polar solvents, while non-polar molecules tend to be more soluble in non-polar solvents. We used this concept to extract the pigments from plants in our research project a few weeks ago.
- Microwaves will react with polar molecules differently than non-polar molecules.
Procedures
Molecular Polarity
We will use the following PhET simulation to better understand when a molecule is polar vs non-polar. Note that this section is graded based on completion, NOT on accuracy, so please complete it in the way that helps you learn the most.
Note: for the linear molecules below, if you see a tiny molecular dipole when you don’t expect there to be a molecular dipole, it’s probably because you don’t quite have the 3 atoms in a perfect line. Try adjusting atom A a little (a few degrees) to see if the molecular dipole arrow disappears.
- Select the “Three Atoms” part of the simulation (you might have to click on it twice).
- Uncheck the “Molecular Dipole” box in the top right corner of your screen.
- Click and drag on atom A to give a linear molecule and set the electronegativities of A and C to high and keep B in the middle as seen below.
- In the “Prediction” column on your short report, predict which atoms have partial negative charges (δ-), partial positive charges (δ+), what bond dipoles it has and what the overall molecular dipole looks like (if it has one). If you don’t think the molecule has an overall molecular dipole, write “no molecular dipole” underneath the molecule.
- Check the “Bond Dipoles”, “Molecular Dipole”, and “Partial Charges” boxes at the top right of your screen to check your answer. (Do NOT turn the “Electric Field” on.)
- If your prediction is different from the correct answer, put the correct partial negative charges, partial positive charges, bond dipoles and overall molecular dipole in the “Actual” column on your short report. Note that this simulation puts the molecular dipole arrow next to the molecule, but I want you to put the molecular dipole arrow on top of the molecule so that the “+” part of the arrow is at the center of the overall partial positive charge and the pointy end of the arrow is at the center of the overall partial negative charge.
- If you consistently get your predictions wrong, please ask your instructor for help.
- Uncheck the “Bond Dipoles”, “Molecular Dipole”, and “Partial Charges” boxes at the top right of your screen, then set the electronegativity of A and C to low and keep B in the middle as seen below. Repeat steps 4-6 for this new molecule.
- Uncheck the “Bond Dipoles”, “Molecular Dipole”, and “Partial Charges” boxes at the top right of your screen, then set the electronegativity of C to high, keeping A at low and B in the middle as seen below. Repeat steps 4-6 for this new molecule.
- Uncheck the “Bond Dipoles”, “Molecular Dipole”, and “Partial Charges” boxes at the top right of your screen, then set the electronegativity all of the atoms to high as seen below. Repeat steps 4-6 for this new molecule.
- Uncheck the “Bond Dipoles”, “Molecular Dipole”, and “Partial Charges” boxes at the top right of your screen, then click and drag on atom A to give a bent molecule with a 110°-120° bond angle and set the electronegativities of A and C to high and B to the middle as seen below. Repeat steps 4-6 for this new molecule.
- Uncheck the “Bond Dipoles”, “Molecular Dipole”, and “Partial Charges” boxes at the top right of your screen, then set the electronegativity of A and C to low and keep B in the middle as seen below. Repeat steps 4-6 for this new molecule.
- Uncheck the “Bond Dipoles”, “Molecular Dipole”, and “Partial Charges” boxes at the top right of your screen, then set the electronegativity of C to high, keeping A at low and B in the middle as seen below. Repeat steps 4-6 for this new molecule.
- Uncheck the “Bond Dipoles”, “Molecular Dipole”, and “Partial Charges” boxes at the top right of your screen, then set the electronegativity all of the atoms to high as seen below. Repeat steps 4-6 for this new molecule.
- If you got no more than 3 of your predictions wrong, describe the process you used to determine the partial charges, bond dipoles and molecular dipole. If you got more than 3 predictions wrong, you may skip this question.
Building VSEPR Models Using Model Kits
- For molecules with trigonal bipyramidal electron geometries, it is vital that you put the bonds in the correct holes corresponding to the correct type of electron position!
- If there are double bonds, you must use the appropriate number of flexible bonds rather than treat this as a single bond.
- It is hard to distinguish between the phosphorus and nitrogen atoms; their colors are similar. Be sure to count the number of holes for these.
- It is expected that many students would find it hard to do all of these by themselves initially. While you are expected to make a good-faith effort to solve the problems by yourself first, please don’t hesitate to ask your instructor questions if you don’t understand something or you get stuck.
This is an exercise designed to allow you to gain experience in visualizing molecules as three-dimensional entities. A number of the consequences of three-dimensional structure will be explored at the same time as notation is developed to present three-dimensional structures in diagrams and drawings.
You will construct some “ball and stick” molecular models using a kit. The different balls and sticks typically represent different bonds and atoms, as listed below.
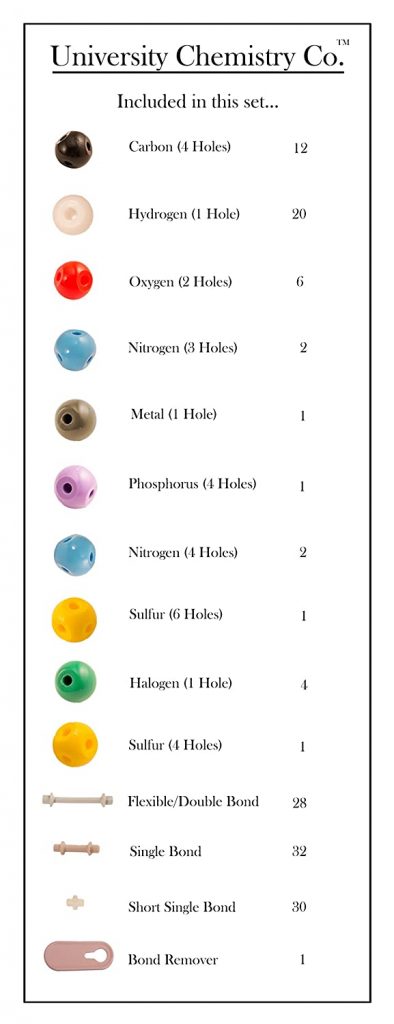
For this part, follow the instructions below for each molecule given on pages 4-6 of your short report. Be sure to use the molecular geometry table above to help you determine the electronic and molecular geometries.
- Draw the Lewis structure (including lone pairs) for the molecule at the top of each column of the given table on pages 4-6 of your short report (ignore bond angles, this is just to determine the connectivity).
- Determine if all of the atoms have an octet/duet. If not, double check whether that atom is allowed to not have an octet. If it is, continue. If it isn’t, draw a new Lewis structure that gives it an octet.
- Give the name of the electronic geometry of the central atom (remember that electronic geometry looks at the arrangement of the electrons around the central atom).
- Based on the Lewis structure, build the model for the structure. For any atom that violates the octet rule, you need to use a model of an atom with the same number of holes as bonds/lone pairs on that atom (double bond = 2 bonds, triple bond = 3 bonds) (e.g. 1 double bond, 1 single bond and 2 lone pairs means 5 holes are necessary). Remember that lone pairs should always be put in an equatorial position, not an axial position. For Al, use an atom model that has 5 holes, but only use the equatorial holes, not the axial holes. After building the model, redraw the Lewis structure with the correct 3D molecular geometry (i.e. accurate bond angles) for the molecule using solid wedges and dashed/hashed wedges as mentioned above. Include all lone pairs present on all atoms in the molecule. It is generally best to put as many bonds in the plane of the paper as possible. Hint: if the molecule doesn’t have 90-degree bond angles, then you shouldn’t draw a right angle between any bonds.
- AFTER you have drawn the correct 3D structure, give the name of the molecular geometry of the central atom (remember that molecular geometry looks only at the arrangement of the atoms around the central atom).
- If the molecular geometry is NOT linear, draw another 3D structure of the molecule from a different perspective that has a different number of wedges/dashes/lines. For example, if your first perspective has 2 lines and 2 wedges/dashes, try drawing a perspective that has 1 line and 3 wedges/dashes or 0 lines and 4 wedges/dashes. To help you with this, take the 3D model you made of the molecule and rotate it ~90 in any direction. If you are still having trouble doing this, ask your instructor for help and they will demonstrate.
- If the molecular geometry IS linear, redraw the molecule from a different perspective with the same number of wedges/dashes/lines.
- Draw all BOND dipoles present on the 2nd perspective of the molecule that you drew for question 6. If there are no bond dipoles on the molecule, write “non-polar” beneath the molecule instead.
- Determine if the overall molecule is polar or non-polar as discussed above. If it is polar, draw the molecular dipole on the 1st perspective you drew for question 4. If it is non-polar, write “non-polar” beneath the molecule instead.
Once you have finished pages 4-6 in your short report, complete the questions on page 7 of your short report.
Acknowledgments
This experiment is based on a similar experiment developed for Chemistry 105 at Fort Hays State University, Kansas and an activity by Nicole Hughes at Bishop Foley Catholic High School in Madison Heights, Michigan.