15 Action potentials
Learning Objectives
After reading this section, you should be able to-
- Compare and contrast graded potentials and action potentials, with particular attention to their locations in the neuron and the ions and ion channels involved in each.
- Label a voltage-versus-time diagram of an action potential with the ions involved in each phase, the direction of their movement across the membrane, and the terms depolarize, repolarize, and hyperpolarize.
- Describe the physiological process involved in the conduction (propagation) of an action potential, including the types and locations of the ion channels involved.
Resting membrane potential describes the steady state of the cell, which is a dynamic process balancing ions leaking down their concentration gradient and ions being pumped back up their concentration gradient. Without any outside influence, the resting membrane potential will be maintained. To get an electrical signal started, the membrane potential has to become more positive.
In the previous chapter, we introduced graded potentials—localized changes in membrane potential that occur in the dendrites or cell body. Graded potentials vary in strength, diminish with distance, and may be excitatory or inhibitory. If a graded potential is strong enough to bring the membrane at the axon hillock to threshold, an action potential is triggered. In contrast to graded potentials, action potentials are all-or-none signals that travel long distances without losing strength.
This starts with the opening of voltage-gated Na+ channels in the neuron membrane. Because the concentration of Na+ is higher outside the cell than inside the cell by a factor of 10, ions will rush into the cell, driven by both the chemical and electrical gradients. Because sodium is a positively charged ion, as it enters the cell it will change the relative voltage immediately inside the cell membrane. The resting membrane potential is approximately -70 mV, so the sodium cation entering the cell will cause the membrane to become less negative. This is known as depolarization, meaning the membrane potential moves toward zero (becomes less polarized). The concentration gradient for Na+ is so strong that it will continue to enter the cell even after the membrane potential has become zero, so that the voltage immediately around the pore then begins to become positive.
As the membrane potential reaches +30 mV, slower voltage-gated potassium channels open in the membrane. An electrochemical gradient acts on K+, as well. As K+ starts to leave the cell, taking a positive charge with it, the membrane potential begins to move back toward its resting voltage of -70 mV. This is called repolarization.
Repolarization returns the membrane potential to the -70 mV value of the resting potential, but overshoots that value. Potassium ions reach equilibrium when the membrane voltage is below -70 mV, so a period of hyperpolarization occurs while the K+ channels are open. Those K+ channels are slightly delayed in closing, accounting for this short overshoot.
What has been described here is the action potential, which is presented as a graph of voltage over time in Figure 15.1. It is the electrical signal that nervous tissue generates for communication. The change in the membrane voltage from -70 mV at rest to +30 mV at the end of depolarization is a 100-mV change.
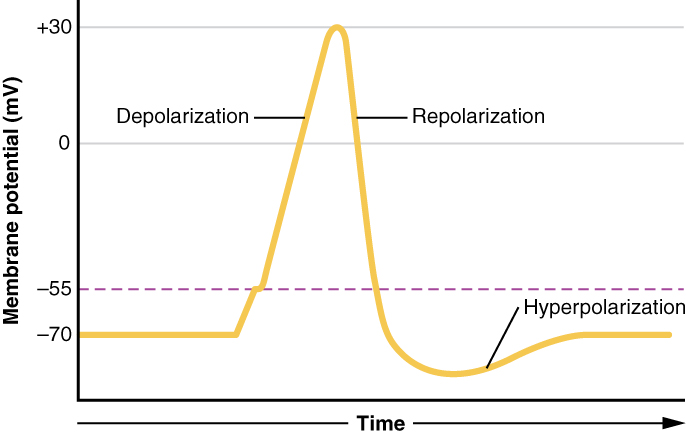
Action Potential Initiation
The membrane potential will stay at the resting voltage until something changes. To begin an action potential, the membrane potential must change from the resting potential of approximately -70mV to the threshold voltage of -55mV. Once the cell reaches threshold, voltage-gated sodium channels open and begin the predictable membrane potential changes described above as an action potential. Any stimulus that depolarizes the membrane to -55 mV or beyond will cause a large number of channels to open and an action potential will be initiated. Any sub-threshold depolarization that does not change the membrane potential to -55 mV or higher will not reach threshold, and thus will not result in an action potential.
Because of the predictable changes that occur once threshold is reached, the action potential is referred to as “all or none”. This means that either the action potential occurs and is repeated along the entire length of the neuron, or no action potential occurs. A stronger stimulus, which might depolarize the membrane well past threshold, will not make a “bigger” action potential. Either the membrane reaches the threshold and everything occurs as described above, or the membrane does not reach the threshold and nothing else happens. All action potentials peak at the same voltage (+30 mV), so one action potential is not bigger than another. Stronger stimuli will initiate multiple action potentials more quickly, but the individual signals are not bigger.
Voltage-gated Channels
As we have seen, the depolarization and repolarization of an action potential are dependent on two types of channels (the voltage-gated Na+ channel and the voltage-gated K+ channel). The voltage-gated Na+ channel has two gates. One is the activation gate, which opens when the membrane potential crosses -55 mV. The other gate is the inactivation gate, which closes after a specific period of time—on the order of a fraction of a millisecond. When a cell is at rest, the activation gate is closed and the inactivation gate is open. However, when the threshold is reached, the activation gate opens, allowing Na+ to rush into the cell. Timed with the peak of depolarization, the inactivation gate closes. During repolarization, no more sodium can enter the cell. When the membrane potential passes -55 mV again, the activation gate closes. After that, the inactivation gate re-opens, making the channel ready to start the whole process over again. Both gates must be open in order for Na+ to get into the cell.
The voltage-gated K+ channel has only one gate, which is sensitive to a membrane voltage of -50 mV. However, it does not open as quickly as the voltage-gated Na+ channel does. It takes a fraction of a millisecond for the K+ channel to open once that voltage has been reached, which coincides exactly with when the Na+ flow peaks. So voltage-gated K+ channels open just as the voltage-gated Na+ channels are being inactivated. As the membrane potential repolarizes and the voltage passes -50 mV again, the K+ channels begin to close. Potassium continues to leave the cell for a short while and the membrane potential becomes more negative, resulting in the hyperpolarization overshoot. The voltage-gated K+ channels close and the membrane returns to the resting potential because of the ongoing activity of the leak channels and the Na+/K+ ATPase pump.
Once initiated, the action potential travels down the axon toward the axon terminals. In the next chapter, we’ll explore how this signal is propagated along both myelinated and unmyelinated axons and why action potentials only move in one direction.
Adapted from Anatomy & Physiology by Lindsay M. Biga et al, shared under a Creative Commons Attribution-ShareAlike 4.0 International License, chapter 12.
A difference in the amount of a solute in two solutions
the difference in charge between the inside and outside of the cell
A period of time when membrane potential is become less negative
A period of time when membrane potential returns to its original negative state
A period of time when membrane potential is becoming more negative
a change in membrane potential that occurs due to the opening and closing of voltage-gated ion channels on the cell membrane
a gate that is voltage-dependent
a gate that is time-dependent
cellular active transport pump responsible for transporting sodium out of a cell and moving potassium into the cell