13 Functions and channels of the nervous system
Learning Objectives
After reading this section, you should be able to-
- Describe the general functions of the nervous system
- Differentiate between the motor (efferent) and sensory (afferent) components of the nervous system
- List the major ion channels of neurons and describe them as leak (leakage or passive) or voltage-gated channels, mechanically gated channels, or ligand-gated (chemically-gated) channels, and identify where they typically are located on a neuron
- Describe the physiological basis of the resting membrane potential (RMP) in a neuron including the ion channels involved, the relative ion concentrations, and the electrochemical gradient.
The Central and Peripheral Nervous Systems
The picture you have in your mind of the nervous system probably includes the brain, the nervous tissue contained within the cranium, and the spinal cord, the extension of nervous tissue within the vertebral column. Additionally, the nervous tissue that reach out from the brain and spinal cord to the rest of the body- (nerves) -are also part of the nervous system. We can anatomically divide the nervous system into two major regions: the central nervous system (CNS) is the brain and spinal cord, and the peripheral nervous system (PNS) is the nerves (Figure 13.1). The brain is contained within the cranial cavity of the skull, and the spinal cord is contained within the vertebral canal of the vertebral column. The peripheral nervous system is so named because it is in the periphery—meaning beyond the brain and spinal cord.
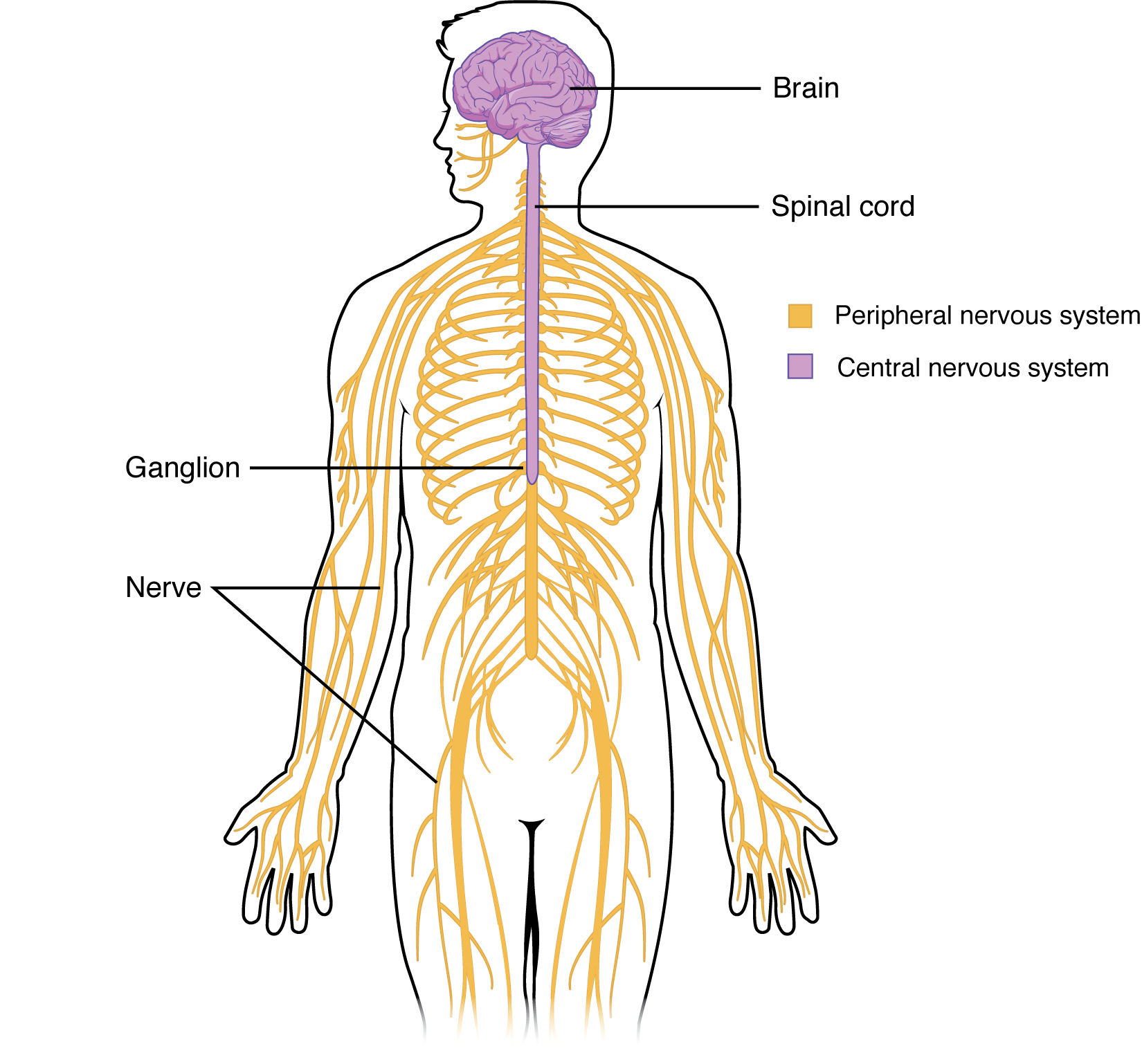
Functional Divisions of the Nervous System
In addition to the anatomical divisions listed above, the nervous system can also be divided on the basis of its functions. The nervous system is involved in receiving information about the environment around us (sensory functions, sensation) and generating responses to that information (motor functions, responses) and coordinating the two (integration).
Sensation. Sensation refers to receiving information about the environment, either what is happening outside (ie: heat from the sun) or inside the body (ie: heat from muscle activity). These sensations are known as stimuli (singular = stimulus) and different sensory receptors are responsible for detecting different stimuli. Sensory information travels towards the CNS through the PNS nerves in the specific division known as the afferent (sensory) branch of the PNS. When information arises from sensory receptors in the skin, skeletal muscles, or joints, it is transmitted to the CNS using somatic sensory neurons; when information arises from sensory receptors in the blood vessels or internal organs, it is transmitted to the CNS using visceral sensory neurons.
Integration. Stimuli that are detected by sensory structures are communicated to the nervous system where information is processed. In the CNS, information from some stimuli is compared with, or integrated with, information from other stimuli or memories of previous stimuli. Then, a motor neuron is activated to initiate a response from the effector organ. This process during which sensory information is processed and a motor response generated is called integration (Figure 13.2).
Response. The nervous system produces a response in effector organs (such as muscles or glands) due to the sensory stimuli. The motor (efferent) branch of the PNS carries signals away from the CNS to the effector organs. When the effector organ is a skeletal muscle, the neuron carrying the information is called a somatic motor neuron; when the effector organ is cardiac or smooth muscle or glandular tissue, the neuron carrying the information is called an autonomic motor neuron. Voluntary responses are governed by somatic motor neurons and involuntary responses are governed by autonomic motor neurons, which are discussed in the next section.
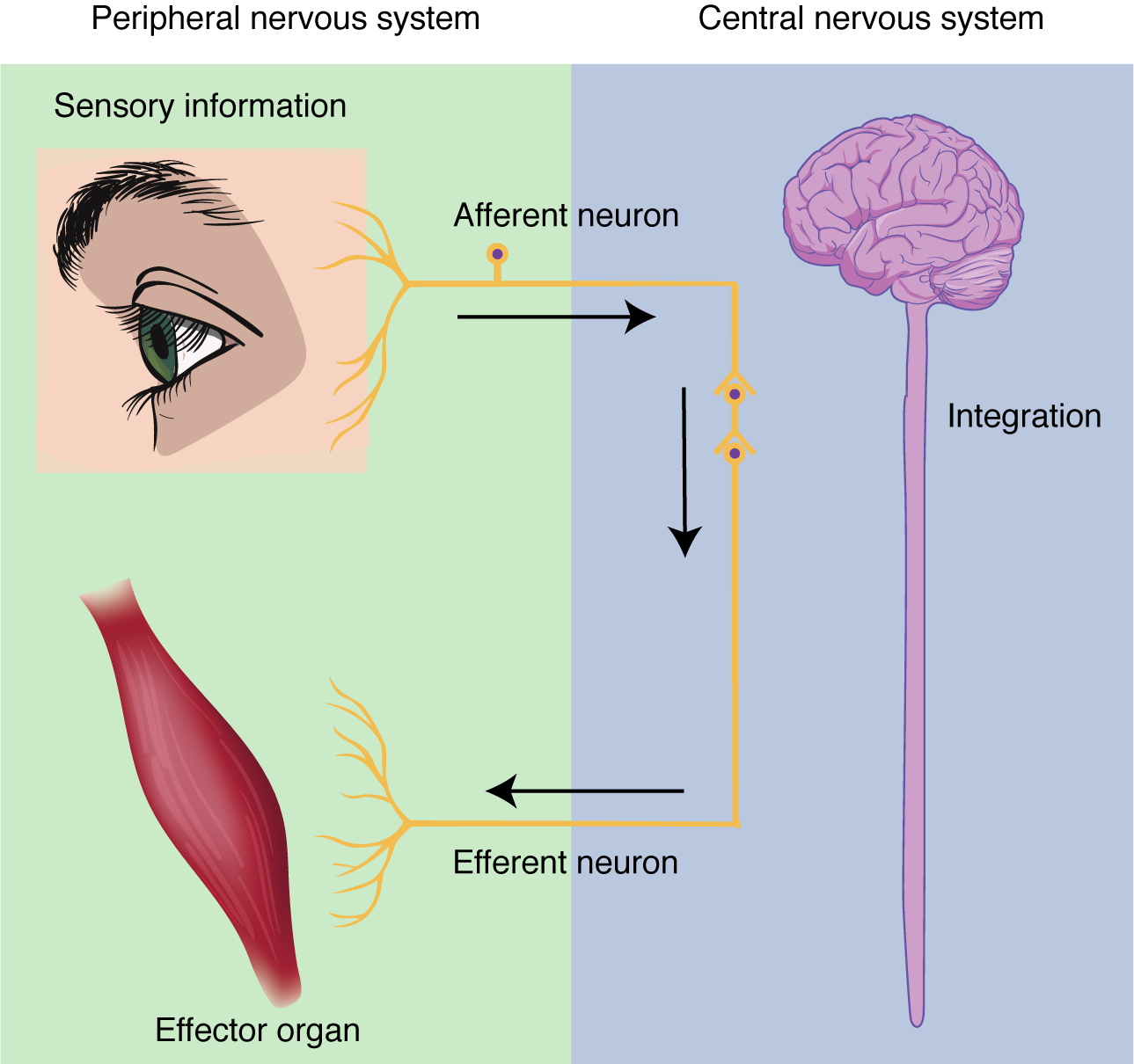
The functions of the nervous system—sensation, integration, and response—depend on the functions of the neurons underlying these pathways. To understand how neurons are able to communicate, it is necessary to describe the role of an excitable membrane in generating these signals. The basis of this process is the action potential. An action potential is a predictable change in membrane potential that occurs due to the opening and closing of voltage gated ion channels on the cell membrane.
Electrically Active Cell Membranes
Most cells in the body make use of charged particles (ions) to create electrochemical charge across the cell membrane. In a prior chapter, we described how muscle cells contract based on the movement of ions across the cell membrane. For skeletal muscles to contract, due to excitation–contraction coupling, they require input from a neuron. Both muscle and nerve cells make use of a cell membrane that is specialized for signal conduction to regulate ion movement between the extracellular fluid and cytosol.
As you learned in the chapter on cells, the cell membrane is primarily responsible for regulating what can cross the membrane. The cell membrane is a phospholipid bilayer, so only substances that can pass directly through the hydrophobic core can diffuse through unaided. Charged particles, which are hydrophilic, cannot pass through the cell membrane without assistance (Figure 13.3). Specific transmembrane channel proteins permit charged ions to move across the membrane. Several passive transport channels, as well as active transport pumps, are necessary to generate a transmembrane potential, and an action potential. Of special interest is the carrier protein referred to as the sodium/potassium pump that uses energy to move sodium ions (Na+) out of a cell and potassium ions (K+) into a cell, thus regulating ion concentration on both sides of the cell membrane.
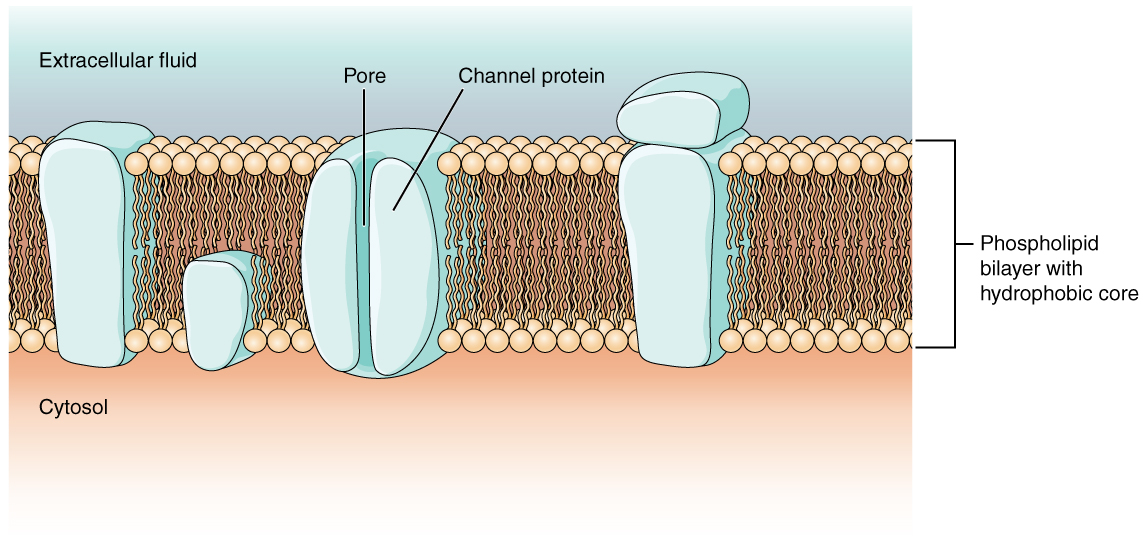
The sodium/potassium pump requires energy in the form of adenosine triphosphate (ATP), so it is also referred to as an ATPase pump. As was explained in the cell chapter, the concentration of Na+ is higher outside the cell than inside, and the concentration of K+ is higher inside the cell than outside. Therefore, this pump works against the concentration gradients for sodium and potassium ions, which is why it requires energy. The Na+/K+ ATPase pump maintains these important ion concentration gradients.
Ion channels are pores that allow specific charged particles to cross the membrane in response to an existing electrochemical gradient. Proteins are capable of spanning the cell membrane, including its hydrophobic core, and can interact with charged ions because of the varied properties of amino acids found within specific regions of the protein channel. Hydrophobic amino acids are found in the regions that are adjacent to the hydrocarbon tails of the phospholipids, where as hydrophilic amino acids are exposed to the fluid environments of the extracellular fluid and cytosol. Additionally, ions will interact with the hydrophilic amino acids, which will be selective for the charge of the ion. Channels for cations (positive ions) will have negatively charged side chains in the pore. Channels for anions (negative ions) will have positively charged side chains in the pore. The diameter of the channel’s pore also impacts the specific ions that can pass through. Some ion channels are selective for charge but not necessarily for size. These nonspecific channels allow cations—particularly Na+, K+, and Ca2+—to cross the membrane, but exclude anions.
Some ion channels do not allow ions to freely diffuse across the membrane, but are gated instead. A ligand-gated channel opens because a molecule, or ligand, binds to the extracellular region of the channel (Figure 13.4). These channels are usually found on the dendrites of a cell.
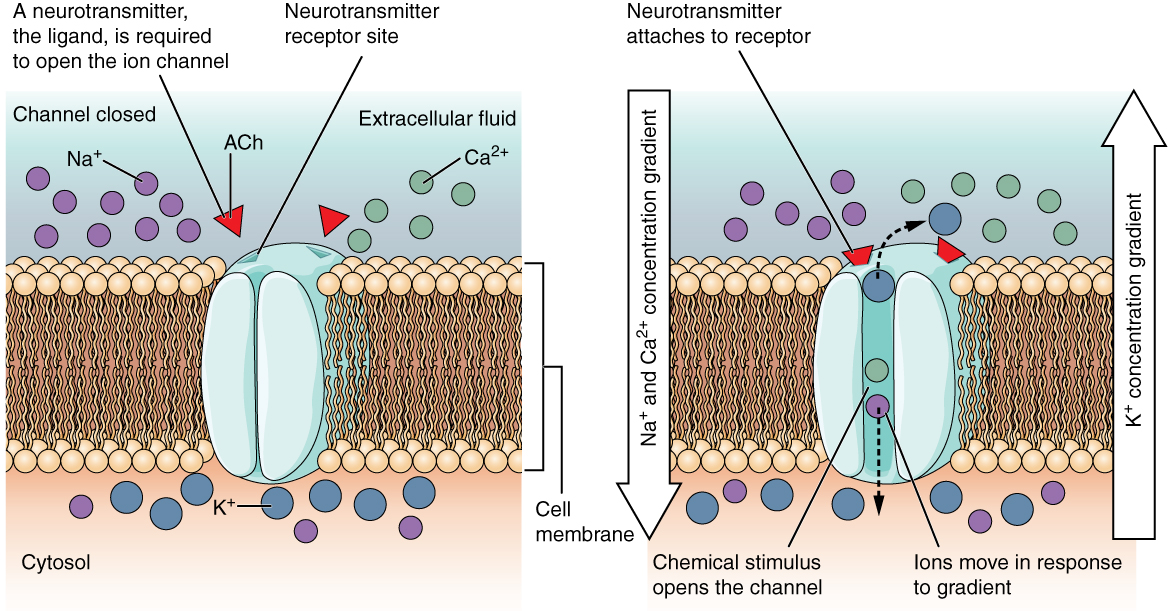
A mechanically-gated channel opens because of a physical distortion of the cell membrane. Many channels associated with the sense of touch are mechanically-gated. For example, as pressure is applied to the skin, mechanically-gated channels on the subcutaneous receptors open and allow ions to enter (Figure 13.5).
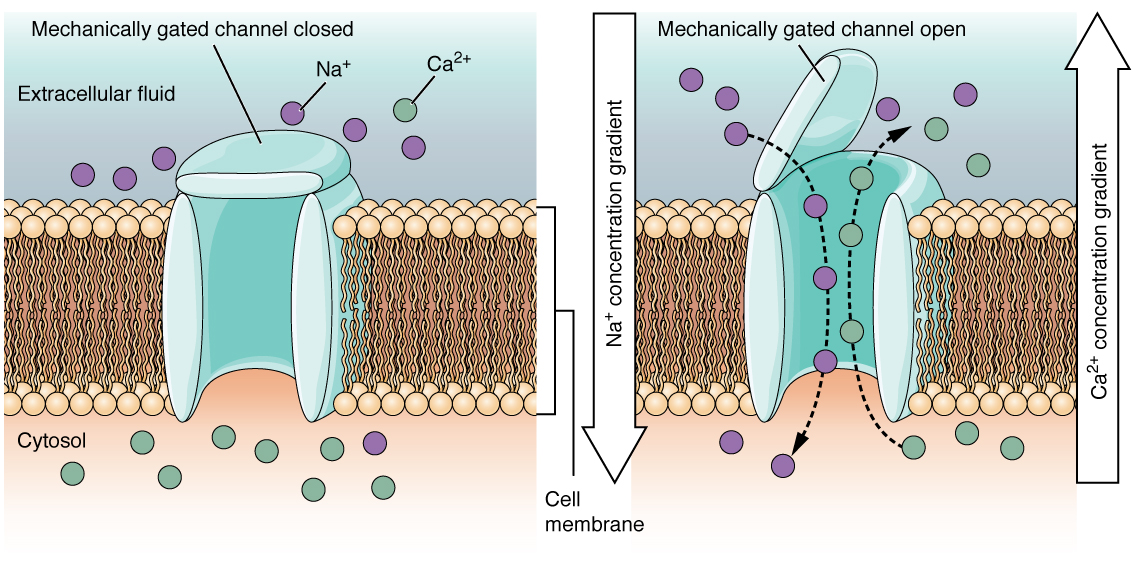
A voltage-gated channel is a channel that responds to changes in the electrical properties of the membrane in which it is embedded. Normally, the inner portion of the membrane is at a negative voltage. When that voltage becomes less negative and reaches a value specific to the channel, it opens and allows ions to cross the membrane (Figure 13.6).
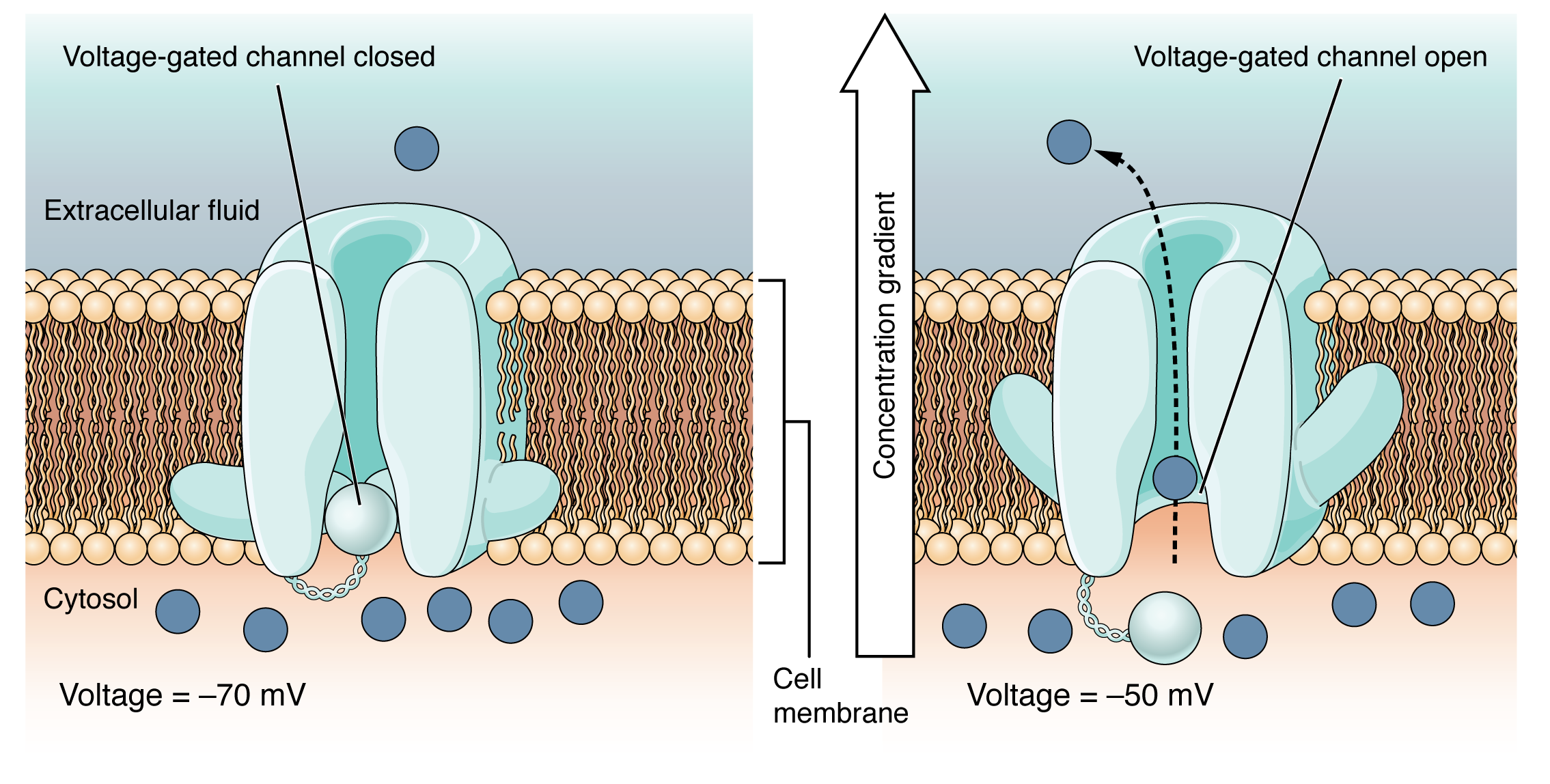
A leak channel is randomly gated, meaning that it opens and closes at random, hence the reference to leaking. There is no actual event that opens the channel; instead, it has an intrinsic rate of switching between the open and closed states. Leak channels contribute to the resting transmembrane voltage of the excitable membrane (Figure 13.7). Voltage-gated and leak channels are generally found along the axon and are involved in maintaining membrane potential, and moving an action potential forward.
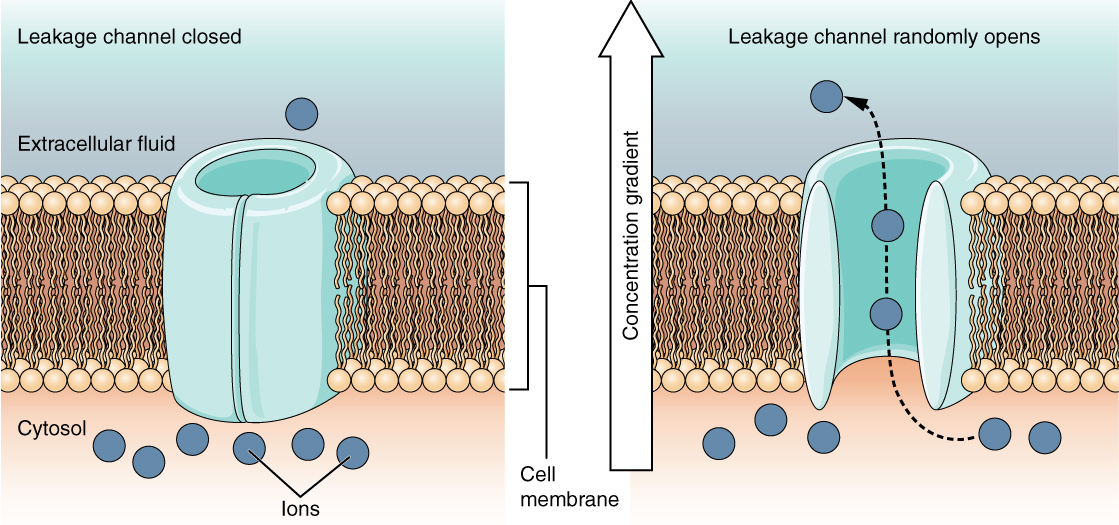
Adapted from Anatomy & Physiology by Lindsay M. Biga et al, shared under a Creative Commons Attribution-ShareAlike 4.0 International License, chapter 12.
organ responsible for controlling all body functions
the column of nerve tissue that extends from the brain into the vertebral canal
bundles of fibers that recieve and send messages between the brain and the body
the brain and the spinal cord
the nervous system outside the brain and spinal cord
the receiving of information about the environment
the processing of sensory information to generate a motor response
a detectable change in the external environment of an organism
the sensory pathway of the peripheral nervous system in which information travels to the central nervous system
sensory division concerned with incoming information from the skin or muscles
sensory division concerned with incoming information from blood vessels and internal organs
a structure that is activated or targeted by the central nervous system in response to a stimulus
the pathway through which motor responses are sent from the central nervous system to the periphery
the voluntary motor pathway; the motor pathway connecting the central nervous system to the muscles and/or skin
the involuntary motor pathway; the motor pathway connecting the central nervous system to smooth muscle, glands, cardiac muscle, and everything else not under voluntary control
a membrane that has the ability to change its permeability to ions and therefore control the movement of ions that carry electrical charges that can cause nerve impulses into and out of the cell
a change in membrane potential that occurs due to the opening and closing of voltage-gated ion channels on the cell membrane
an atom or group of atoms that are electrically charged
fluid portion of the cell made up of the cytosol and cytoplasm; where chemical reactions occur
sheet of two layers of phospholipids, with a hydrophobic interior and hydrophilic exteriors
a substance that repels water
a substance that is attracted to water
cellular active transport pump responsible for transporting sodium out of a cell and moving potassium into the cell
Positively charged ions
Negatively charged ions
active ion channel that requires a molecule to bind to a receptor
a channel that opens due to a physical distortion of the cell membrane
an active ion channel that is activated by changes in voltage along the cell membrane
channel that opens and closes at random