19 G-proteins
Learning Objectives
After reading this section, you should be able to-
- Describe the difference between fast synaptic responses (ion channel [ionotropic] receptors) and slow synaptic responses (second messenger-linked metabotropic receptors).
The important thing to remember about neurotransmitters, and signaling chemicals in general, is that the effect is entirely dependent on the receptor. Neurotransmitters bind to one of two classes of receptors at the cell surface, ionotropic or metabotropic (Figure 19.1). Ionotropic receptors are ligand-gated ion channels, such as the nicotinic receptor for acetylcholine. A metabotropic receptor involves a complex of proteins that result in metabolic changes within the cell. The receptor complex includes the transmembrane receptor protein, a G protein, and an effector protein. The neurotransmitter, referred to as the first messenger, binds to the receptor protein on the extracellular surface of the cell, and the intracellular side of the protein initiates activity of the G protein. The G protein is a guanosine triphosphate (GTP) hydrolase that physically moves from the receptor protein to the effector protein to activate the latter. An effector protein is an enzyme that catalyzes the generation of a new molecule, which acts as the intracellular mediator, or the second messenger.
Different receptors use different second messengers. Two common examples of second messengers are cyclic adenosine monophosphate (cAMP) and inositol triphosphate (IP3). The enzyme adenylate cyclase (an example of an effector protein) makes cAMP, and phospholipase C is the enzyme that makes IP3. Second messengers, after they are produced by the effector protein, cause metabolic changes within the cell. These changes are most likely the activation of other enzymes in the cell. In neurons, the enzymes often modify ion channels, by either opening or closing them. These enzymes can also cause changes in the cell, such as the activation of genes in the nucleus, and therefore the increased synthesis of proteins. In neurons, these kinds of changes are often the basis of stronger connections between cells at the synapse and may be the basis of learning and memory.
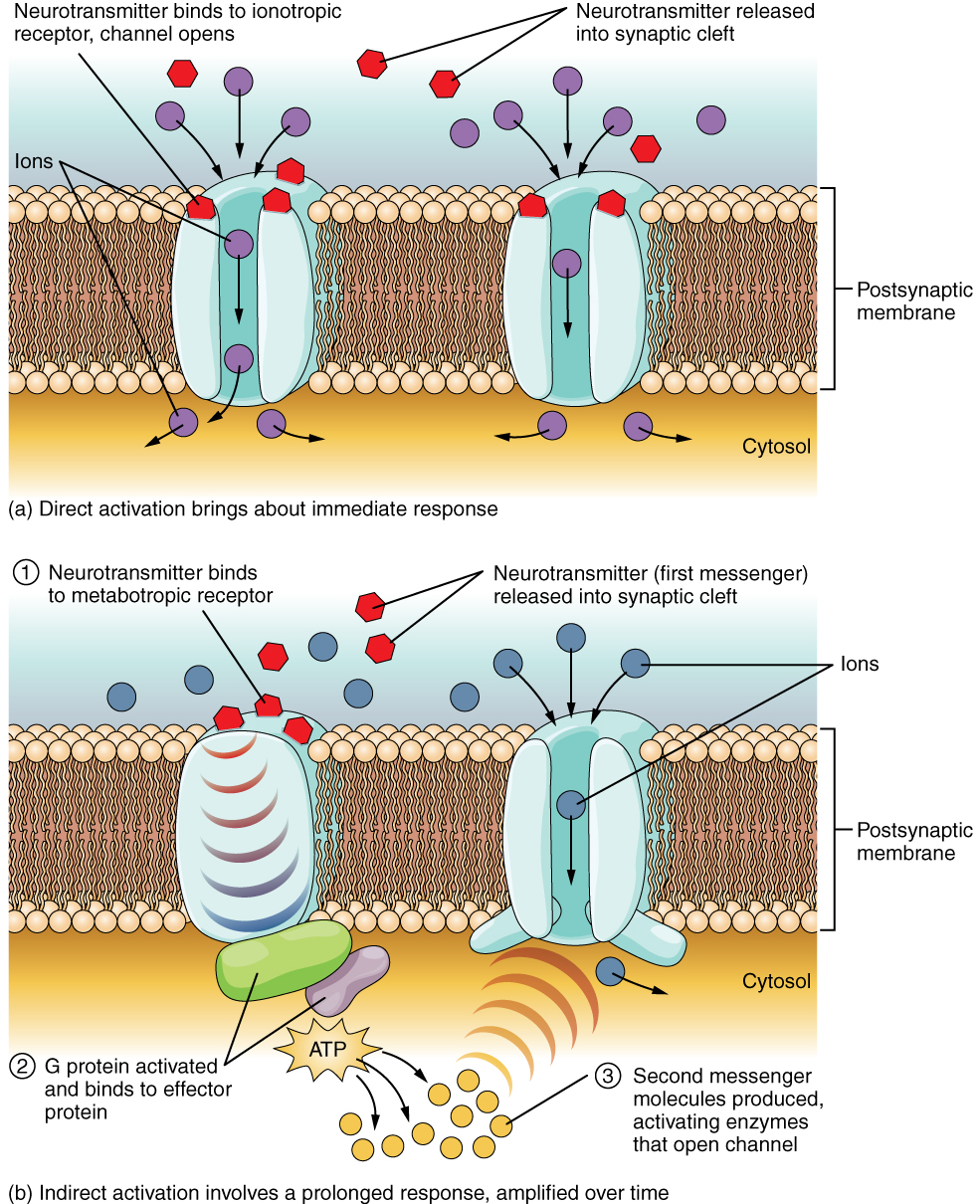
G-alpha S pathway
The G-alpha S pathway is a cellular signaling mechanism that is initiated by the activation of G protein-coupled receptors (GPCRs). In the G-alpha S pathway, the activated G protein’s alpha subunit (G-alpha S) dissociates from the beta and gamma subunits and stimulates the enzyme adenylate cyclase. Adenylate cyclase, in turn, catalyzes the conversion of ATP to cyclic AMP (cAMP). Cyclic AMP is a second messenger that mediates various cellular responses, including the activation of protein kinase A (PKA). PKA then phosphorylates target proteins, initiating a cascade of intracellular events that regulate cell function. The G-alpha S pathway is involved in diverse physiological processes, such as neurotransmission, hormone signaling, and cellular responses to environmental stimuli.
G-alpha I pathway
In the G-alpha I pathway, upon ligand binding to the GPCR, the G protein’s alpha subunit (G-alpha I) is activated and separates from the beta and gamma subunits. The activated G-alpha I inhibits the enzyme adenylate cyclase, preventing the conversion of ATP to cyclic AMP (cAMP). This reduction in cAMP levels leads to the inhibition of protein kinase A (PKA) activity. Consequently, downstream cellular responses are modulated, affecting various physiological processes. The G-alpha I pathway is involved in negative regulation and inhibition of cellular functions, countering the actions of the G-alpha S pathway and contributing to the fine-tuning of cellular responses to different extracellular signals.
G-alpha Q pathway
In the G-alpha Q pathway, upon ligand binding to the GPCR, the G protein’s alpha subunit (G-alpha Q) becomes activated and separates from the beta and gamma subunits. The activated G-alpha Q stimulates the enzyme phospholipase C (PLC). PLC breaks phosphatidylinositol 4,5-bisphosphate (PIP2) into inositol trisphosphate (IP3) and diacylglycerol (DAG). IP3 triggers the release of calcium ions from intracellular stores, leading to various cellular responses, while DAG activates protein kinase C (PKC), initiating a cascade of downstream signaling events. The G-alpha Q pathway is involved in regulating a wide range of cellular processes, including muscle contraction, secretion, and cell proliferation.
Adapted from Anatomy & Physiology by Lindsay M. Biga et al, shared under a Creative Commons Attribution-ShareAlike 4.0 International License, chapter 12.
fast acting ligand-gated ion channels
slow acting receptors that allow the activation of other processes within the cell
specialized proteins with the ability to bind guanosine triphosphate (GTP) and guanosine diphosphate (GDP)
an enzyme that catalyzes the generation of a new molecule