26 Hearing
Learning Objectives
After reading this section, you should be able to-
- Classify the receptor cells for hearing based on the type of stimulus (i.e., modality).
- Trace the path of sound from the external ear to the inner ear, including where sound is amplified.
- Explain the process by which an action potential is generated at the spiral organ (of Corti).
- Explain how the structures of the ear enable differentiation of pitch, intensity (loudness), and localization of sounds.
Hearing, or audition, is the transduction of sound waves into a neural signal that is made possible by the structures of the ear (Figure 26.1). The large, fleshy structure on the lateral aspect of the head is known as the auricle. Some sources will also refer to this structure as the pinna, though that term is more appropriate for a structure that can be moved, such as the external ear of a cat. The C-shaped curves of the auricle direct sound waves toward the auditory canal. The canal enters the skull through the external auditory meatus of the temporal bone. At the end of the auditory canal is the tympanic membrane, or ear drum, which vibrates after it is struck by sound waves. The auricle, ear canal, and tympanic membrane are often referred to as the external ear. The middle ear consists of a space spanned by three small bones called the ossicles. The three ossicles are the malleus, incus, and stapes, which are Latin names that roughly translate to hammer, anvil, and stirrup. The malleus is attached to the tympanic membrane and articulates with the incus. The incus, in turn, articulates with the stapes. The stapes is then attached to the inner ear, where the sound waves will be transduced into a neural signal. The middle ear is connected to the pharynx through the Eustachian tube, which helps equilibrate air pressure across the tympanic membrane. The tube is normally closed but will pop open when the muscles of the pharynx contract during swallowing or yawning.
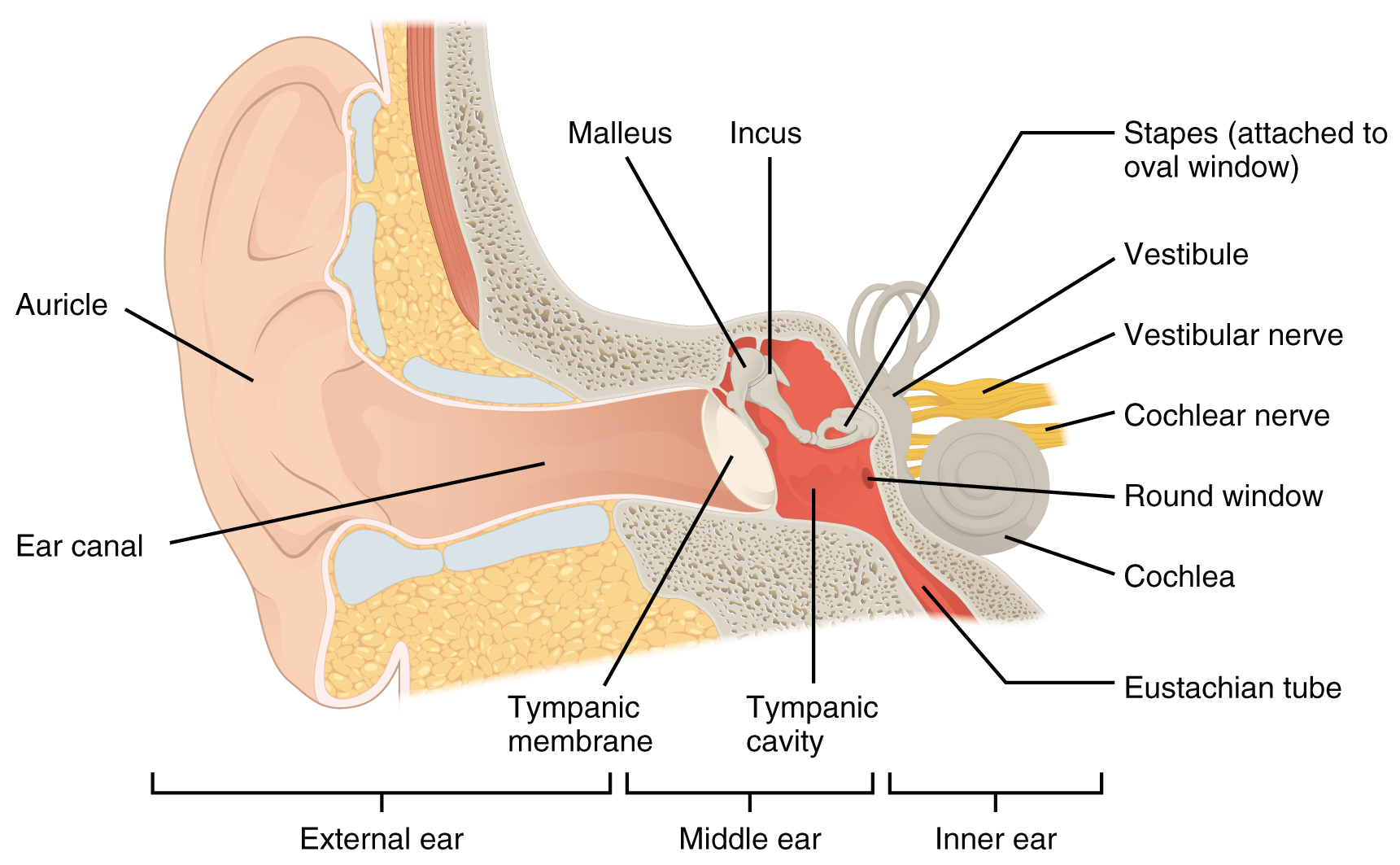
The inner ear is often described as a bony labyrinth, as it is composed of a series of canals embedded within the temporal bone. It has two separate regions, the cochlea and the vestibule, which are responsible for hearing and balance, respectively. The neural signals from these two regions are relayed to the brain stem through separate fiber bundles. However, these two distinct bundles travel together from the inner ear to the brain stem as the vestibulocochlear nerve. Sound is transduced into neural signals within the cochlear region of the inner ear, which contains the sensory neurons of the spiral ganglia. These ganglia are located within the spiral-shaped cochlea of the inner ear. The cochlea is attached to the stapes through the oval window.
The oval window is located at the beginning of a fluid-filled tube within the cochlea called the scala vestibuli. The scala vestibuli extends from the oval window, traveling above the cochlear duct, which is the central cavity of the cochlea that contains the sound-transducing neurons. At the uppermost tip of the cochlea, the scala vestibuli curves over the top of the cochlear duct. The fluid-filled tube, now called the scala tympani, returns to the base of the cochlea, this time traveling under the cochlear duct. The scala tympani ends at the round window, which is covered by a membrane that contains the fluid within the scala. As vibrations of the ossicles travel through the oval window, the fluid of the scala vestibuli and scala tympani moves in a wave-like motion. The frequency of the fluid waves match the frequencies of the sound waves (Figure 26.2). The membrane covering the round window will bulge out or pucker in with the movement of the fluid within the scala tympani.
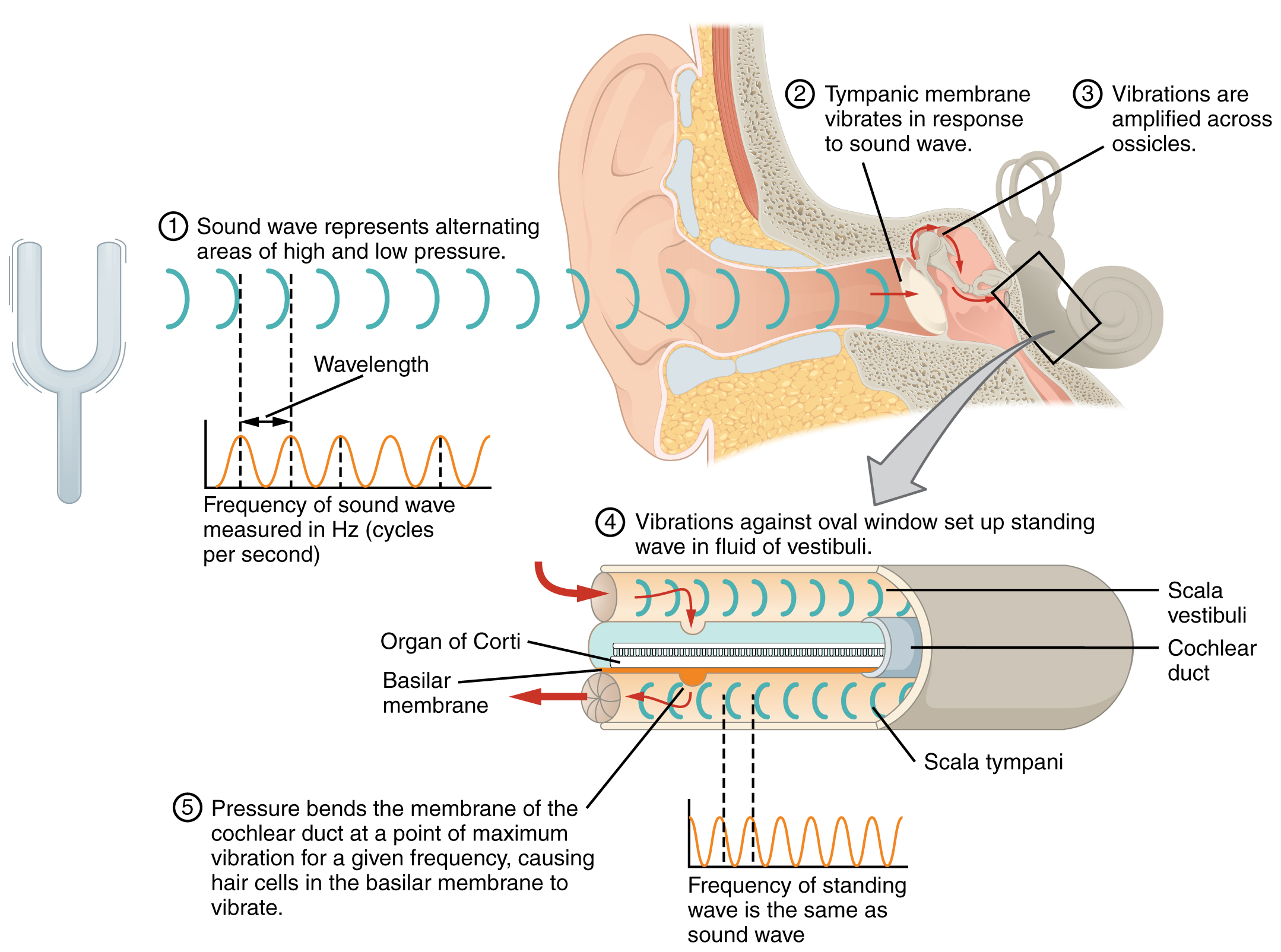
A cross-sectional view of the cochlea shows that the scala vestibuli and scala tympani run along both sides of the cochlear duct (Figure 26.3). The cochlear duct contains several organs of Corti, which tranduce the wave motion of the two scala into neural signals. The organs of Corti lie on top of the basilar membrane, which is the side of the cochlear duct located between the organs of Corti and the scala tympani. As the fluid waves move through the scala vestibuli and scala tympani, the basilar membrane moves at a specific spot, depending on the frequency of the waves. Higher frequency waves move the region of the basilar membrane that is close to the base of the cochlea. Lower frequency waves move the region of the basilar membrane that is near the tip of the cochlea.
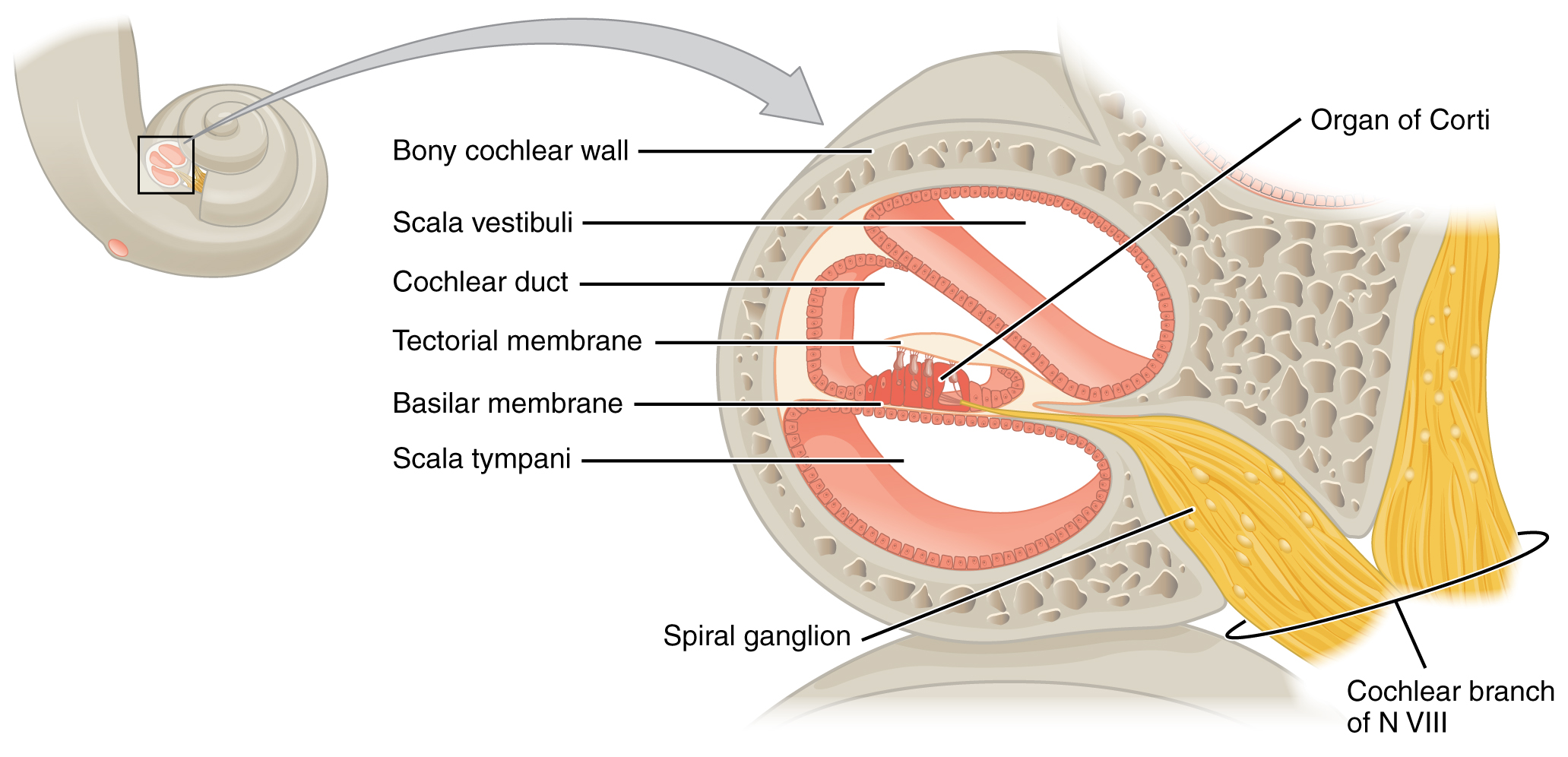
The organs of Corti contain hair cells, which are named for the hair-like stereocilia extending from the cell’s apical surfaces (Figure 26.4). The stereocilia are an array of microvilli-like structures arranged from tallest to shortest. Protein fibers tether adjacent hairs together within each array, such that the array will bend in response to movements of the basilar membrane. The stereocilia extend up from the hair cells to the overlying tectorial membrane, which is attached medially to the organ of Corti. When the pressure waves from the scala move the basilar membrane, the tectorial membrane slides across the stereocilia. This bends the stereocilia either toward or away from the tallest member of each array. When the stereocilia bend toward the tallest member of their array, tension in the protein tethers open ion channels in the hair cell membrane. This will depolarize the hair cell membrane, triggering nerve impulses that travel down the afferent nerve fibers attached to the hair cells. When the stereocilia bend toward the shortest member of their array, the tension on the tethers slackens and the ion channels close. When no sound is present, and the stereocilia are standing straight. A small amount of tension still exists on the tethers, keeping the membrane potential of the hair cell slightly depolarized.
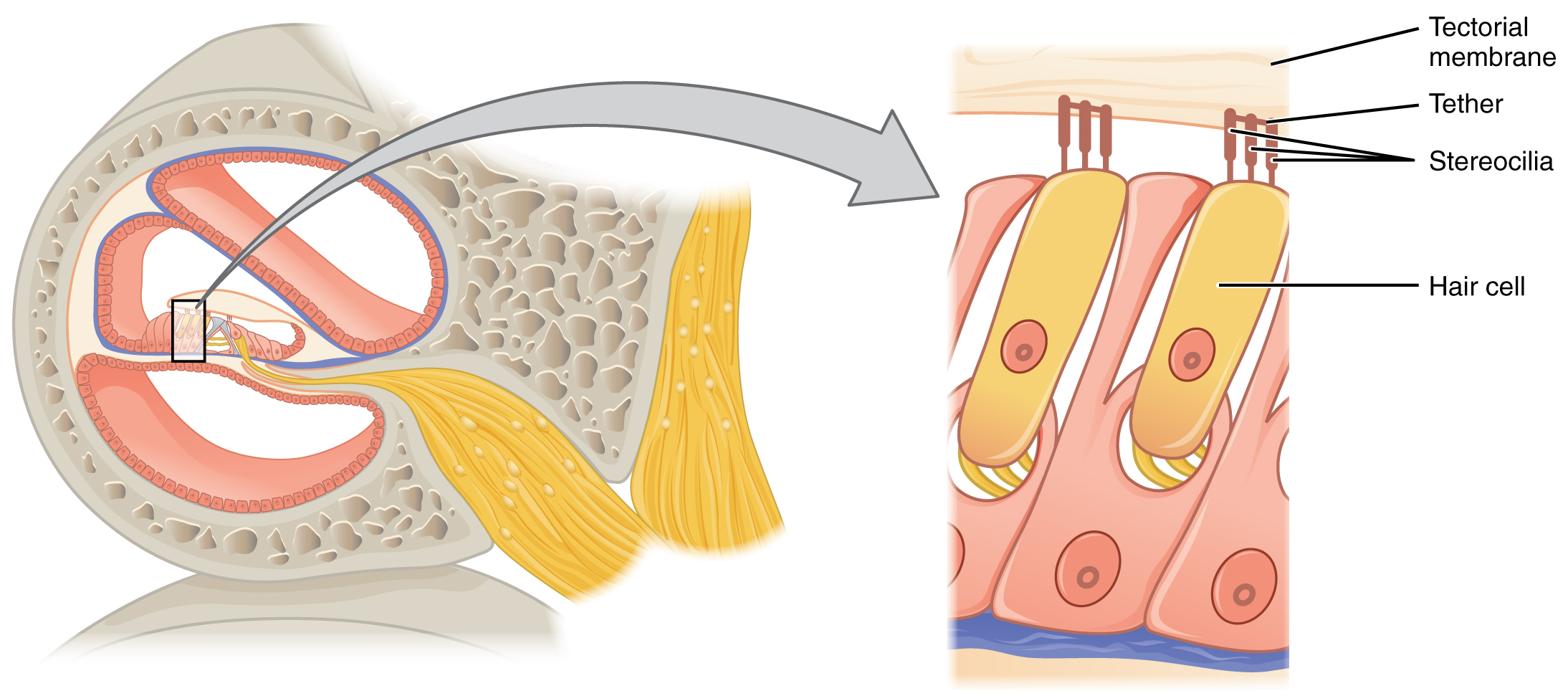
As stated above, a given region of the basilar membrane will only move if the incoming sound is at a specific frequency. Because the tectorial membrane only moves where the basilar membrane moves, the hair cells in this region will also only respond to sounds of this specific frequency. Therefore, as the frequency of a sound changes, different hair cells are activated all along the basilar membrane. The cochlea encodes auditory stimuli for frequencies between 20 and 20,000 Hertz, which is the range of sound that human ears can detect. The unit of Hertz (Hz) measures the frequency of sound waves in terms of cycles produced per second. Frequencies as low as 20 Hz are detected by hair cells at the apex, or tip, of the cochlea. Frequencies in the higher ranges of 20 KHz are encoded by hair cells at the base of the cochlea, closer to the round and oval windows (Figure 26.5). Most auditory stimuli contain a mixture of sounds at a variety of frequencies and intensities (represented by the amplitude of the sound wave). The hair cells along the length of the cochlear duct, which are each sensitive to a particular frequency, allow the cochlea to separate auditory stimuli by frequency, just as a prism separates visible light into its component colors.
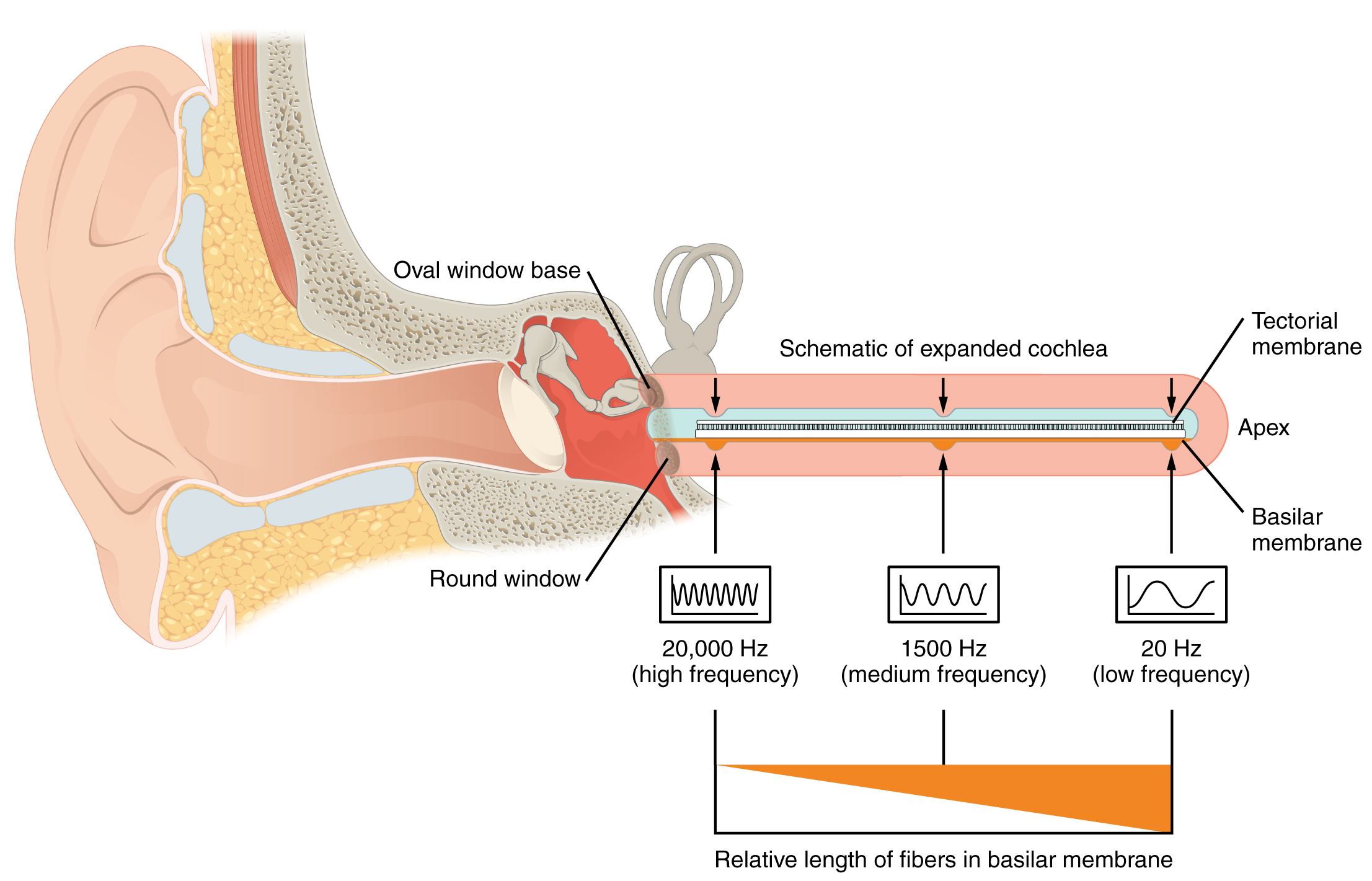
Central Processing of Hearing Information
The sensory pathway for audition travels along the vestibulocochlear nerve, which synapses with neurons in the cochlear nuclei of the superior medulla. Within the brain stem, input from either ear is combined to extract location information from the auditory stimuli. Whereas the initial auditory stimuli received at the cochlea strictly represent the frequency—or pitch—of the stimuli, the locations of sounds can be determined by comparing information arriving at both ears.
Sound localization is a feature of central processing in the auditory nuclei of the brain stem. Sound originating from a specific location will arrive at each ear at different times, unless the sound is directly in front of the listener. If the sound source is slightly to the left of the listener, the sound will arrive at the left ear microseconds before it arrives at the right ear (Figure 26.6). Also, the sound will be slightly louder in the left ear than in the right ear because some of the sound waves reaching the opposite ear are blocked by the head. These differences in timing and volume allow the brain to interpret where the sound is originating from.
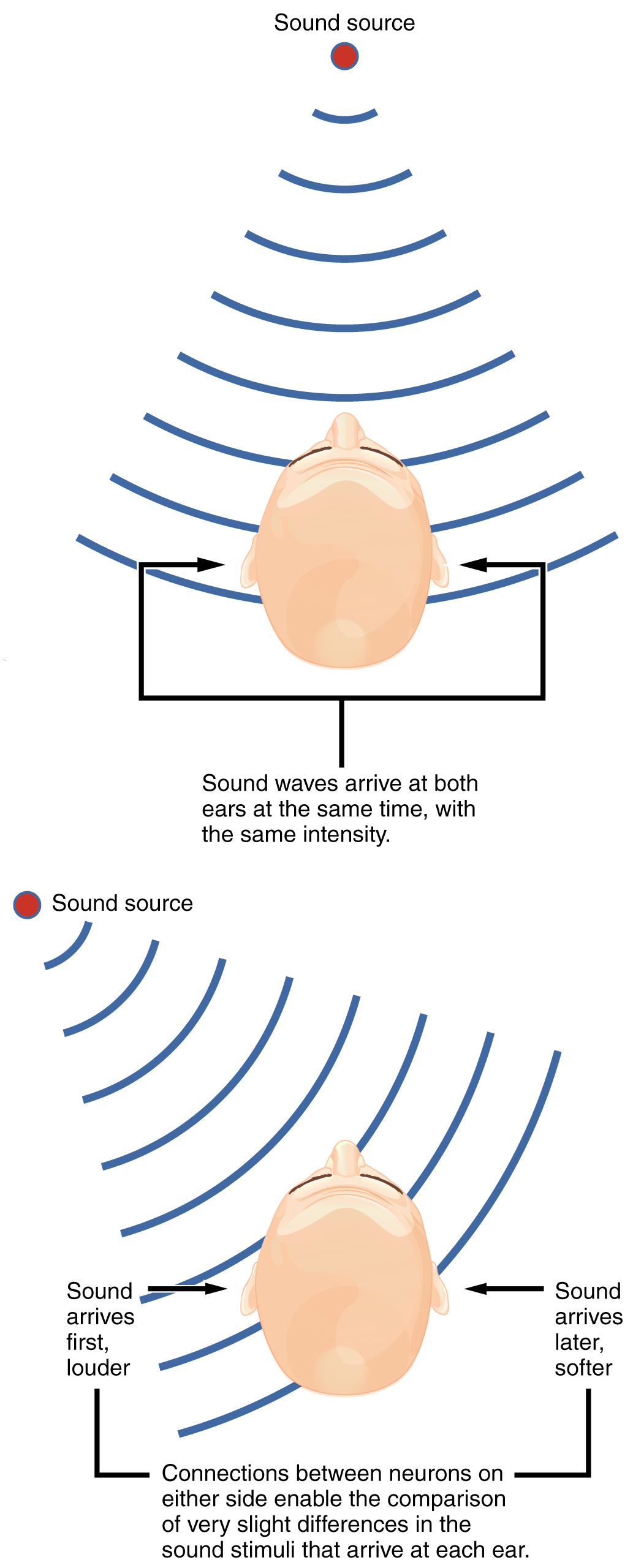
Auditory processing continues on to a nucleus in the midbrain called the inferior colliculus. Axons from the inferior colliculus project to two locations, the thalamus and the superior colliculus. The medial geniculate nucleus of the thalamus receives the auditory information and then projects that information to the auditory cortex in the temporal lobe of the cerebral cortex. The superior colliculus receives input from the visual and somatosensory systems, as well as the ears, to initiate stimulation of the muscles that turn the head and neck toward the auditory stimulus.
Adapted from Anatomy & Physiology by Lindsay M. Biga et al, shared under a Creative Commons Attribution-ShareAlike 4.0 International License, chapter 15.
the sensation of hearing
outer or external ear
ear drum; thin, circular tissue responsible for separating the outer and middle ear
visible part of the ear, made up of the auricle, ear canal, and tympanic membrane
air-filled membrane within the temporal lobe and posterior to the tympanic membrane, containing the ossicles
three small bones within the middle ear responsible for transmitting air vibrations from the tympanic membrane to the inner ear
one of three ossicles within the middle ear responsible for transmitting air vibrations to the incus; hammer-shaped
one of three ossicles within the middle ear responsible for transmitting air vibrations from the malleus to the stapes; anvil-shaped
one of three ossicles within the middle ear responsible for transmitting air vibrations from the incus to the oval window; stirrup-shaped
inner-most part of the ear responsible for converting sound vibrations into electrical signals, made up of the cochlea, semicircular canals, and vestibule
auditory tube; canal connecting the middle ear to the pharynx, responsible for equalizing air pressure in the middle ear
fluid-filled, spiral-shaped cavity within the inner ear containing nerve endings responsible for transmitting sound information to the brain
group of bipolar sensory neuron cell bodies located in the inner ear responsible for transmitting sound information to the brain through their axons
intersection between the middle ear and the cochlea of the inner ear through direct contact with the stapes of the middle ear
perilymph-filled cavity within the cochlea responsible for transmitting sound waves to the scala tympani
endolymph-filled central cavity of the cochlea of the inner ear containing sound-transducing neurons of the cochlear branch of the vestibulocochlear nerve
perilymph-filled tube below the cochlear duct and connected to the round window
structure through which sound waves leave the inner ear
spiral organs; structure within the inner ear resting on the basilar membrane of the cochlear duct containing hair cells that have stereocilia; responsible for transducing wave motion of the two scala into neural signals
structure separating the scala tympani from the cochlear duct
hair-like projections making up the spiral organ which respond to fluid movement from the cochlea
acellular membrane located in the cochlea of the inner ear
paired structure in the midbrain responsible for integrated sound localization and relaying of auditory information from the inner ear traveling to the auditory cortex
paired structure in the midbrain responsible for processing visual, somatosensory input to control head and eye movements
nucleus within the thalamus, between the inferior colliculus and temporal lobe, responsible for receiving auditory information from the inferior colliculus and projecting it to the auditory cortex in the temporal lobe