25 Vision
Learning Objectives
After reading this chapter, you should be able to-
- Trace the path of light as is passes through the eye to the retina, and describe which structures are responsible for refracting the light rays.
- Trace the signal pathway from the retina through the optic nerve, optic chiasm, optic tract, and to the various parts of the brain.
- Explain how the optical system of the eye creates an image on the retina.
- Describe phototransduction (i.e., how light activates photoreceptors) and explain the process of light and dark adaptation.
- Compare and contrast the functions and locations of rods and cones.
Vision is the special sense of sight that is based on the transduction of light stimuli received through the eyes. The eyes are located within either orbit in the skull. The bony orbits surround the eyeballs, protecting them and anchoring the soft tissues of the eye (Figure 25.1). The eyelids, with lashes at their leading edges, help to protect the eye from abrasions by blocking particles that may land on the surface of the eye. The inner surface of each lid is a thin membrane known as the palpebral conjunctiva. The conjunctiva extends over the white areas of the eye (the sclera), connecting the eyelids to the eyeball. Tears are produced by the lacrimal gland, located beneath the lateral edges of the nose. Tears produced by this gland flow through the lacrimal duct.
The retina is composed of several layers and contains specialized cells for the initial processing of visual stimuli. The photoreceptors (rods and cones) change their membrane potential when stimulated by light energy. The change in membrane potential alters the amount of neurotransmitter that the photoreceptor cells release onto bipolar cells in the outer synaptic layer. It is the bipolar cell in the retina that connects a photoreceptor to a retinal ganglion cell (RGC) in the inner synaptic layer. There, amacrine cells contribute to retinal processing before an action potential is produced by the RGC. The axons of RGCs, which lie at the innermost layer of the retina, collect at the optic disc and leave the eye as the optic nerve (see Figure 25.1). Because these axons pass through the retina, there are no photoreceptors at the very back of the eye, where the optic nerve begins. This creates a “blind spot” in the retina, and a corresponding blind spot in our visual field.
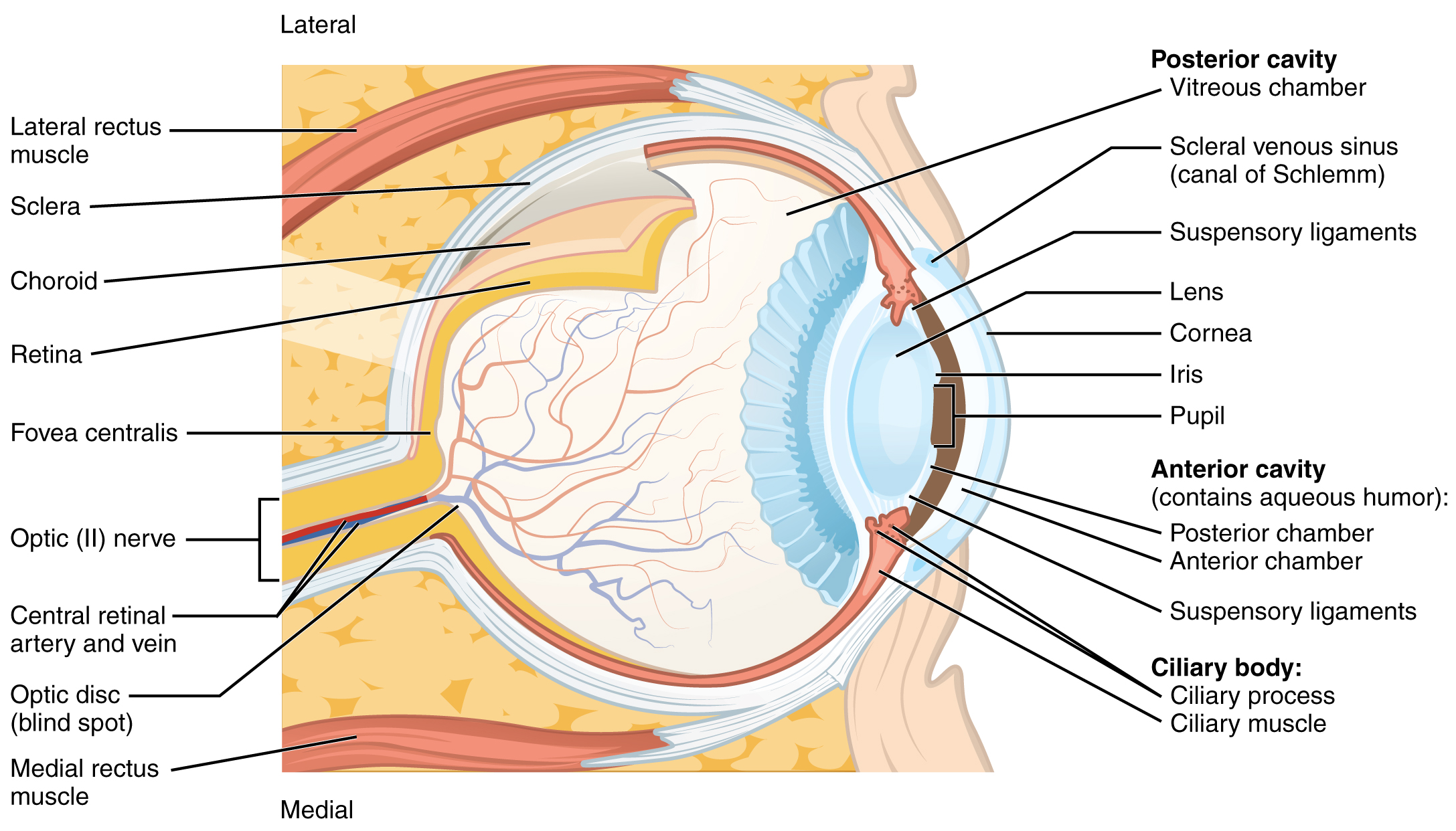
Note that the photoreceptors in the retina are located behind the axons, RGCs, bipolar cells, and retinal blood vessels. A significant amount of light is absorbed by these structures before the light reaches the photoreceptor cells. However, at the exact center of the retina is a small area known as the fovea. At the fovea, the retina lacks supporting cells and blood vessels, and only contains photoreceptors. Therefore, visual acuity, or the sharpness of vision, is greatest at that point. This is because the fovea is where the least amount of incoming light is absorbed by other retinal structures (see Figure 25.1). As one moves in either direction from this central point of the retina, visual acuity drops significantly. In addition, each photoreceptor cell of the fovea is connected to a single RGC. Therefore, this RGC does not have to integrate inputs from multiple photoreceptors, which reduces the accuracy of visual transduction. Toward the edges of the retina, several photoreceptors converge on RGCs (through the bipolar cells) up to a ratio of 50 to 1. The difference in visual acuity between the fovea and peripheral retina is easily evidenced by looking directly at a word in the middle of this paragraph. The visual stimulus in the middle of the field of view falls on the fovea and is in the sharpest focus. Without moving your eyes off that word, notice that words at the beginning or end of the paragraph are not in focus. The images in your peripheral vision are focused by the peripheral retina, and have vague, blurry edges and words that are not as clearly identified. As a result, a large part of the neural function of the eyes is concerned with moving the eyes and head so that important visual stimuli are centered on the fovea.
Light falling on the retina causes chemical changes to pigment molecules in the photoreceptors, ultimately leading to a change in the activity of the RGCs. Photoreceptor cells have two parts, the inner segment and the outer segment (Figure 25.2). The inner segment contains the nucleus and other common organelles of a cell, whereas the outer segment is a specialized region in which photoreception takes place. There are two types of photoreceptors—rods and cones—which differ in the shape of their outer segment. The rod-shaped outer segments of the rod photoreceptor contain a stack of membrane-bound discs that contain the photosensitive pigment rhodopsin. The cone-shaped outer segments of the cone photoreceptor contain their photosensitive pigments in infoldings of the cell membrane. There are three cone photopigments, called opsins, which are each sensitive to a particular wavelength of light. The wavelength of visible light determines its color. The pigments in human eyes are specialized in perceiving three different primary colors: red, green, and blue.
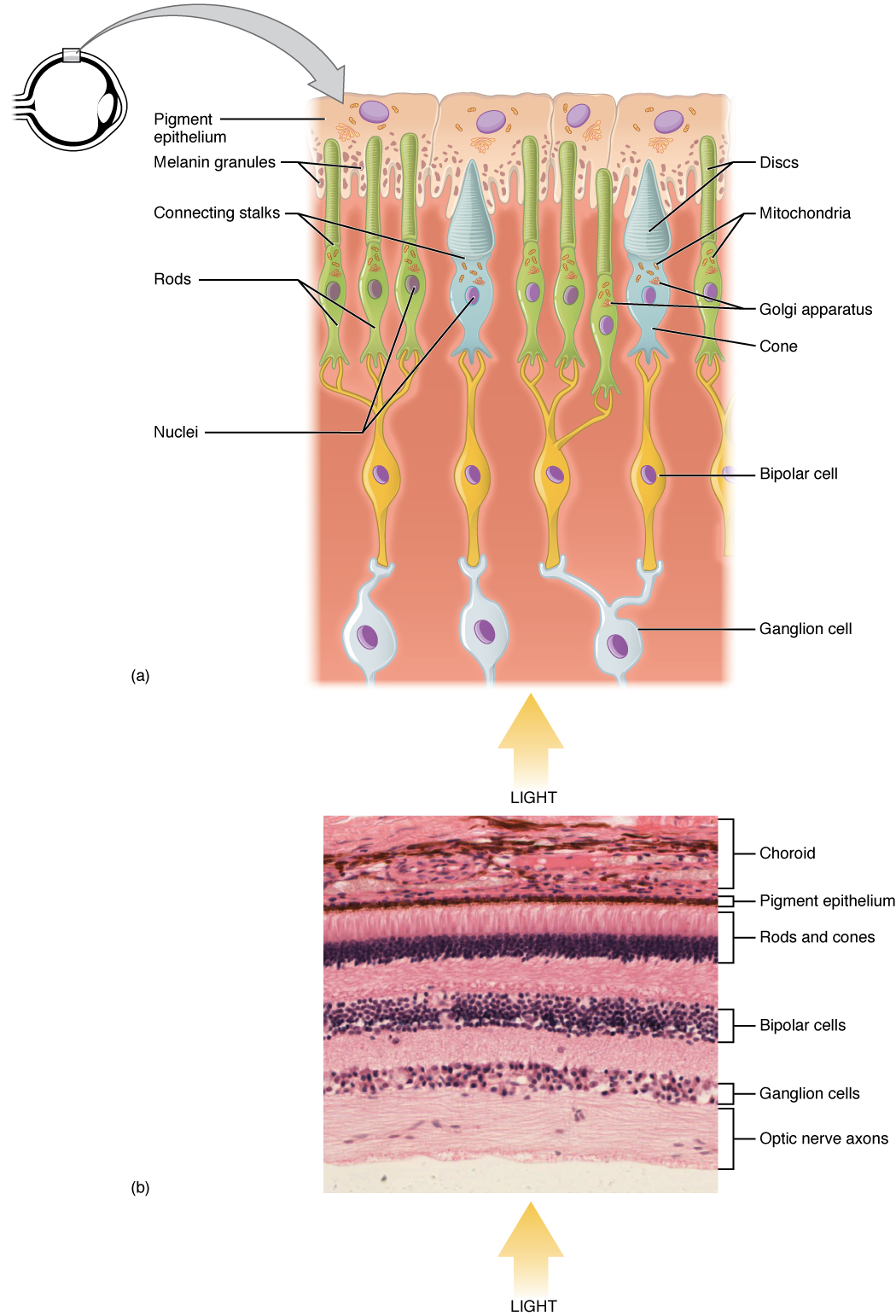
At the molecular level, visual stimuli cause changes in the photopigment molecule that lead to changes in membrane potential of the photoreceptor cell. A single unit of light is called a photon, which is described in physics as a packet of energy with properties of both a particle and a wave. The energy of a photon is represented by its wavelength, with each wavelength of visible light corresponding to a particular color. Visible light is electromagnetic radiation with a wavelength between 380 and 720 nanometers (nm). Wavelengths of electromagnetic radiation longer than 720 nm fall into the infrared range, whereas wavelengths shorter than 380 nm fall into the ultraviolet range. Light with a wavelength of 380 nm is blue, whereas light with a wavelength of 720 nm is dark red. All other colors fall between red and blue at various points along the wavelength scale.
Opsin pigments are actually transmembrane proteins that contain a cofactor known as retinal. Retinal is a hydrocarbon molecule related to vitamin A. When a photon hits retinal, the long hydrocarbon chain of the molecule is biochemically altered. Specifically, photons cause some of the double-bonded carbons within the chain to switch from a cis to a trans conformation. This process is called photoisomerization. Before interacting with a photon, retinal’s flexible double-bonded carbons are in the cis conformation. This molecule is referred to as 11-cis-retinal. A photon interacting with the molecule causes the flexible double-bonded carbons to change to the trans– conformation, forming all-trans-retinal, which has a straight hydrocarbon chain (Figure 25.3).
The shape change of retinal in the photoreceptors initiates visual transduction in the retina. Activation of retinal and the opsin proteins result in activation of a G-protein. The G-protein changes the membrane potential of the photoreceptor cell, which then releases less neurotransmitter into the outer synaptic layer of the retina. Until the retinal molecule is changed back to the 11-cis-retinal shape, the opsin cannot respond to light energy, which is called bleaching. When a large group of photopigments is bleached, the retina will send information as if opposing visual information is being perceived. After a bright flash of light, afterimages are usually seen in negative. The photoisomerization is reversed by a series of enzymatic changes so that the retinal responds to more light energy.
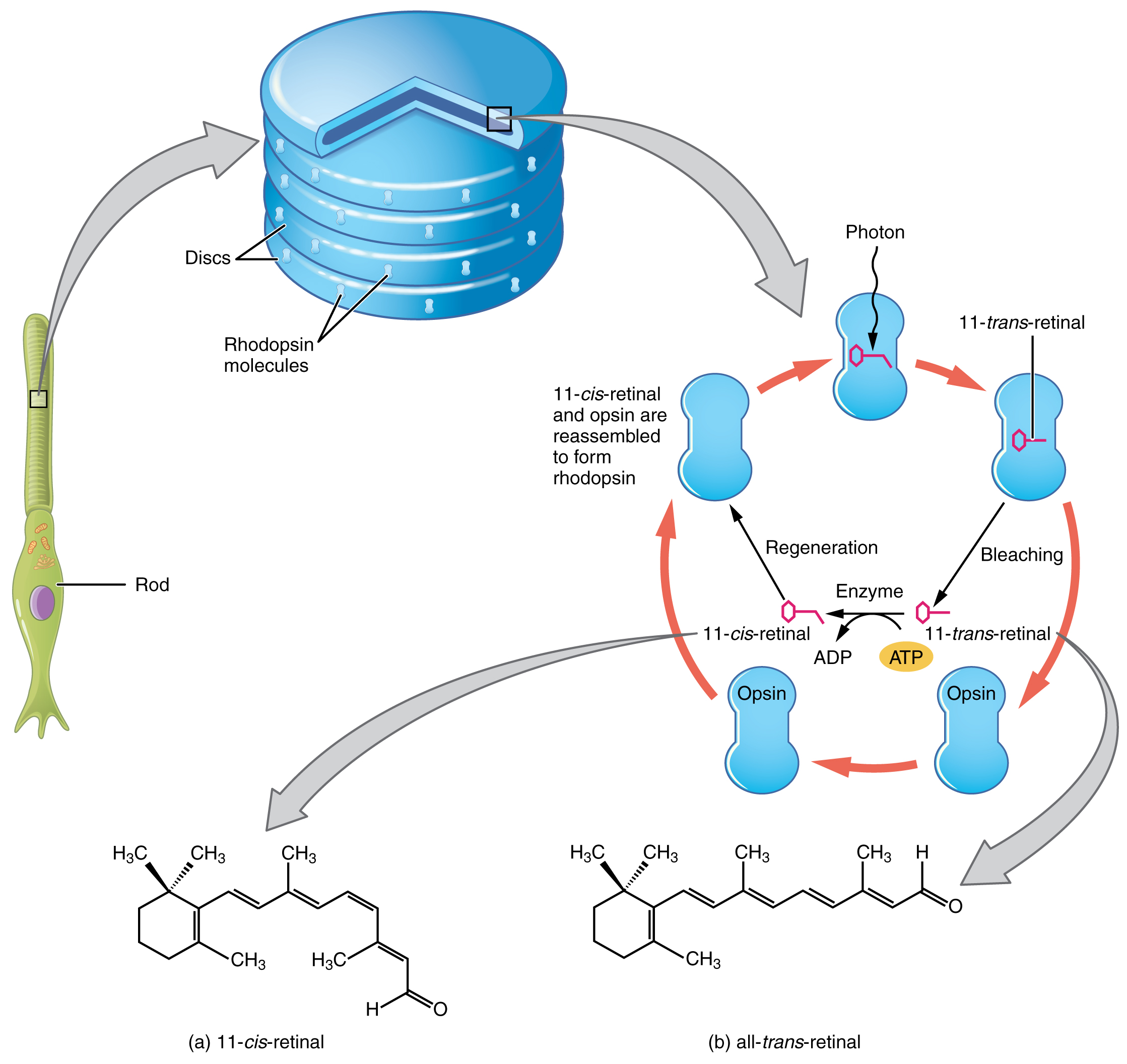
The opsins are sensitive to limited wavelengths of light. Rhodopsin, the photopigment in rods, is most sensitive to light at a wavelength of 498 nm. The three color opsins have peak sensitivities of 564 nm, 534 nm, and 420 nm corresponding roughly to the primary colors of red, green, and blue (Figure 25.4). The absorbance of rhodopsin in the rods is much more sensitive than in the cone opsins; specifically, rods are sensitive to vision in low light conditions, and cones are sensitive to brighter conditions. In normal sunlight, rhodopsin will be constantly bleached while the cones are active. In a darkened room, there is not enough light to activate cone opsins, and vision is entirely dependent on rods. Rods are so sensitive to light that a single photon can result in an action potential from a rod’s corresponding RGC.
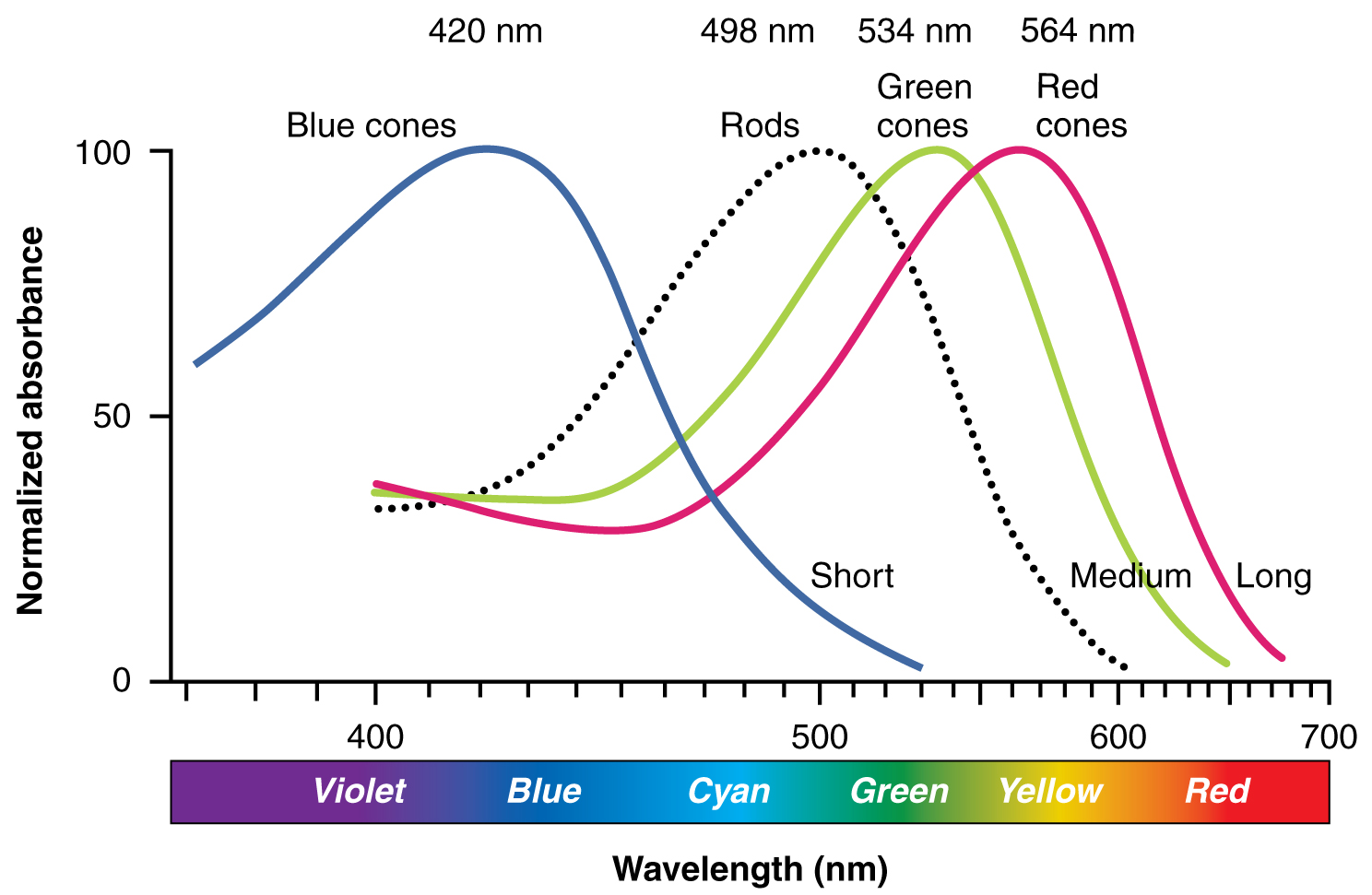
The three types of cone opsins, being sensitive to different wavelengths of light, provide us with color vision. By comparing the activity of the three different cones, the brain can extract color information from visual stimuli. For example, a bright blue light that has a wavelength of approximately 450 nm would activate the “red” cones minimally, the “green” cones marginally, and the “blue” cones predominantly. The relative activation of the three different cones is calculated by the brain, which perceives the color as blue. However, cones cannot react to low-intensity light, and rods do not sense the color of light. Therefore, our low-light vision is—in essence—in grayscale. In other words, in a dark room, everything appears as a shade of gray. If you think that you can see colors in the dark, it is most likely because your brain knows what color something is and is relying on that memory.
Light and Dark Adaptation
Light and dark adaptation are physiological processes that allow the eyes to adjust to varying levels of illumination in the environment. In light adaptation, the transition from darkness to bright light involves the pupil constricting to limit the amount of light entering the eye, and the photoreceptor cells in the retina, particularly the cones, becoming less sensitive to light. This means that it takes a greater amount of light to stimulate these photoreceptors, which prevents overwhelming stimulation of the photoreceptors. Conversely, dark adaptation occurs when transitioning from a bright environment to a dim or dark one. During dark adaptation, the pupils dilate to allow more light to enter the eye, and the sensitivity of the rod cells in the retina increases. This heightened sensitivity maximizes the detection of low levels of light, enabling better vision in low-light conditions. Both light and dark adaptation are crucial for maintaining visual function across a range of lighting conditions, demonstrating the remarkable adaptability of the human visual system.
Adapted from Anatomy & Physiology by Lindsay M. Biga et al, shared under a Creative Commons Attribution-ShareAlike 4.0 International License, chapter 15.
the sensation of sight based on the transduction of light stimuli received through the eyes
the lining of the eyelids
the gland responsible for secreting fluid, or tears, that protects and cleanses the surface of the eye
the tear duct
interneurons in the retina of the eye that receive neural light information from photoreceptor cells
a layer of synapses within the retina
neurons that send information from the retina to the visual processing centers of the central nervous system
a layer of synapses between axons of bipolar cells and dendrites of ganglion cells
interneurons in the retina of the eye
the section at the posterior of the eye where the retina and optic nerve connect, formed by the axons of ganglion cells leaving the eye
also known as cranial nerve II; the cranial nerve that transmits visual information to the central nervous system
area within the retina that only contains photoreceptors
the sharpness of vision
segment of the photoreceptor cell containing the nucleus and other organelles, responsible for cellular protein synthesis and metabolism
segment of photoreceptor cells responsible for phototransduction
photoreceptor cell concentrated near the outer edges of the retina that respond primarily to low light
G-protein-coupled receptor in rod photoreceptors responsible for triggering phototransduction in dim light
photoreceptor cell concentrated near the center of the retina that detect color
G-protein-coupled receptors in cone photoreceptors responsible for triggering phototransduction in particular wavelengths of light
single unit of light
hydrocarbon cofactor molecule in opsin transmembrane proteins
form of isomerization in photoreceptor cells during which light is used to convert one isomer into another